Nanoelectronics Group
We explore the Quantum World using electronic circuits whose size is so small that electrons behave as matter wave. The wave property of electrons gives rise to quantum interference which manifest as oscillations of the conductance versus a phase difference. Their dual particle property is observable in current fluctuations, called quantum shot noise.
We use the best and cleanest quantum conductors known to date:
- 2D electrons confined in high mobility epitaxial GaAs/GaAlAs semi-conductor heterojunctions
- Graphene : an atom thick honeycomb lattice of Carbon atoms which shows amazing properties and where electrons behave as massless quasi-particle obeying the Dirac equation in 2 dimension.
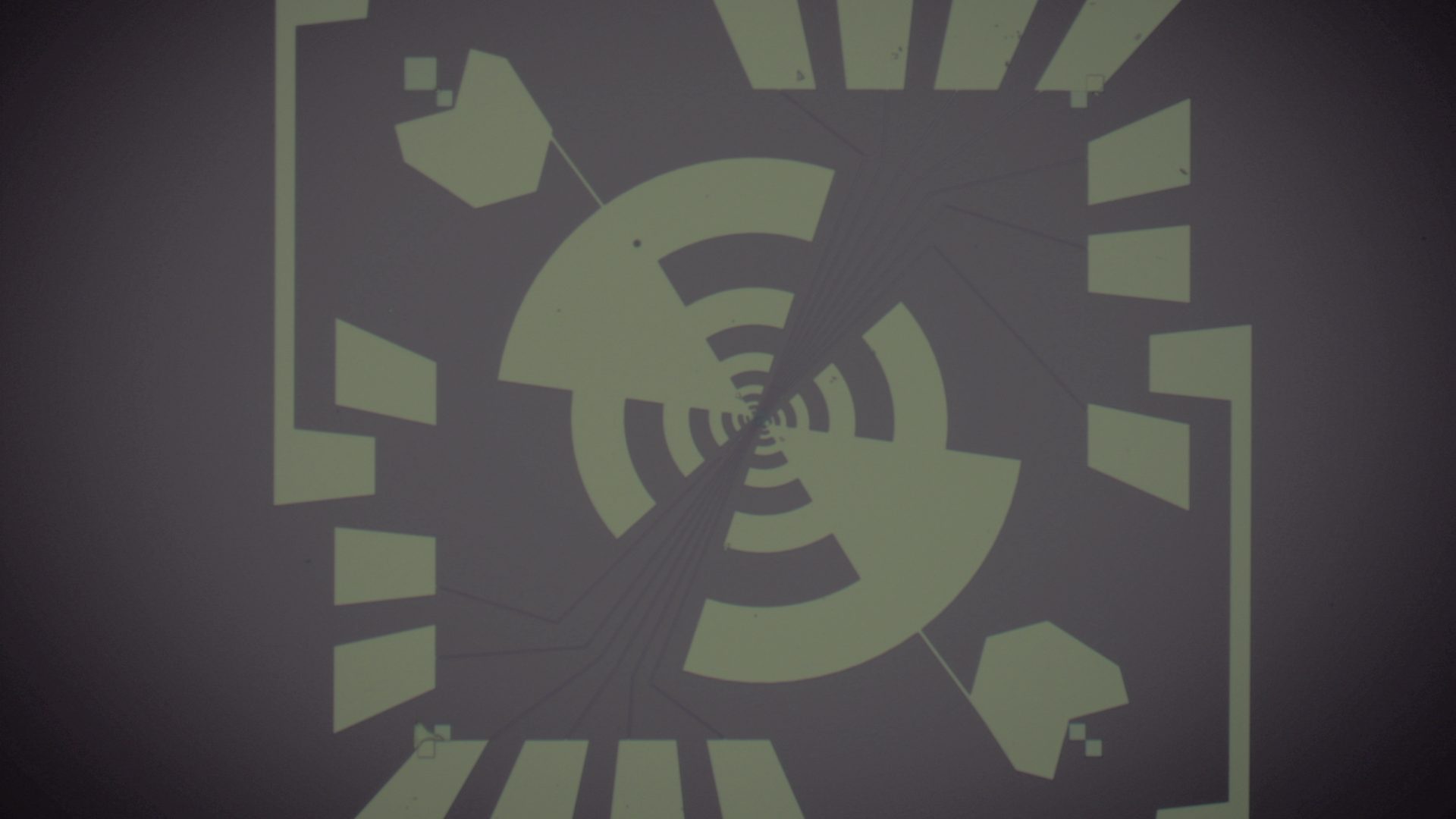
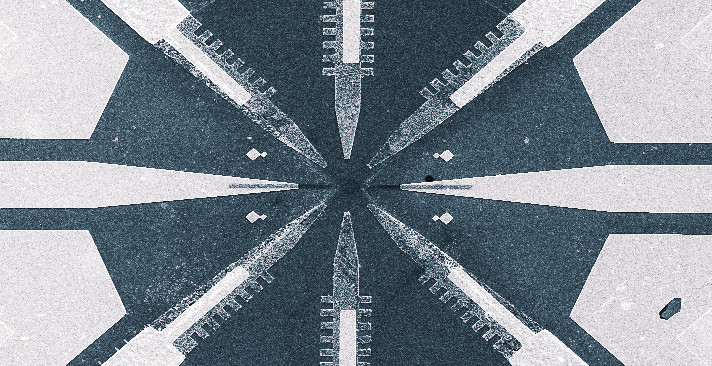
Heat quantum transport in graphene
The one-dimensional, dissipationless edge channels of the quantum Hall effect can be combined with the wave-like nature of electrons at low temperature to perform experiments where the propagation of charges in a quantum circuit mimics the one of light in physical optics experiments. We perform electron optics experiments to probe the coherence properties of charges propagating along quantum Hall edge channels. A striking example of such physics, which we have extensively investigated, are electronic interferences in a Mach-Zehnder geometry, where a beam of electron is split in two, then recombined after a finite propagation length, giving rise to quantum interferences in the electric current.
We are currently investigating the mechanisms leading to energy relaxation of charges propagating along an edge channel, highlighting the strong influence of electron-electron interactions in these systems.
Contact: Patrice Roche
Electron Interferometry in the quantum Hall regime
The ability to obtain ultra-clean graphene (a two-dimensional crystal made of carbon atoms in a honeycomb lattice) samples has recently allowed the observation of intriguing phases of condensed matter in graphene under high magnetic fields. In particular, exotic states of the quantum Hall effect were observed at the Dirac point, where interactions and electronic correlations can either make graphene completely electrically insulating, or give rise to the quantum spin Hall effect. In the latter, the bulk of the two-dimensional crystal is insulating, while electronic current is only carried along the edges of the crystal, with opposite spins propagating in opposite directions. The exact nature of those various states is still not fully understood, as one cannot probe the properties of the insulating regions by usual electron transport measurements.
We propose a new approach to probe those phases, based on the measurement of quantum heat flow carried by chargeless excitations such as spin waves, at very low temperature. Our method consists in connecting the graphene crystal to small metallic electrodes which will be used as heat reservoirs. The temperature of each reservoir will be inferred by ultra-sensitive noise measurements, allowing us to extract the heat flow. The samples will made of graphene encapsulated in hexagonal boron nitride. This technique, which we have recently developed in our lab, allows to obtain large-area, ultra-clean graphene flakes.
Contact: François Parmentier
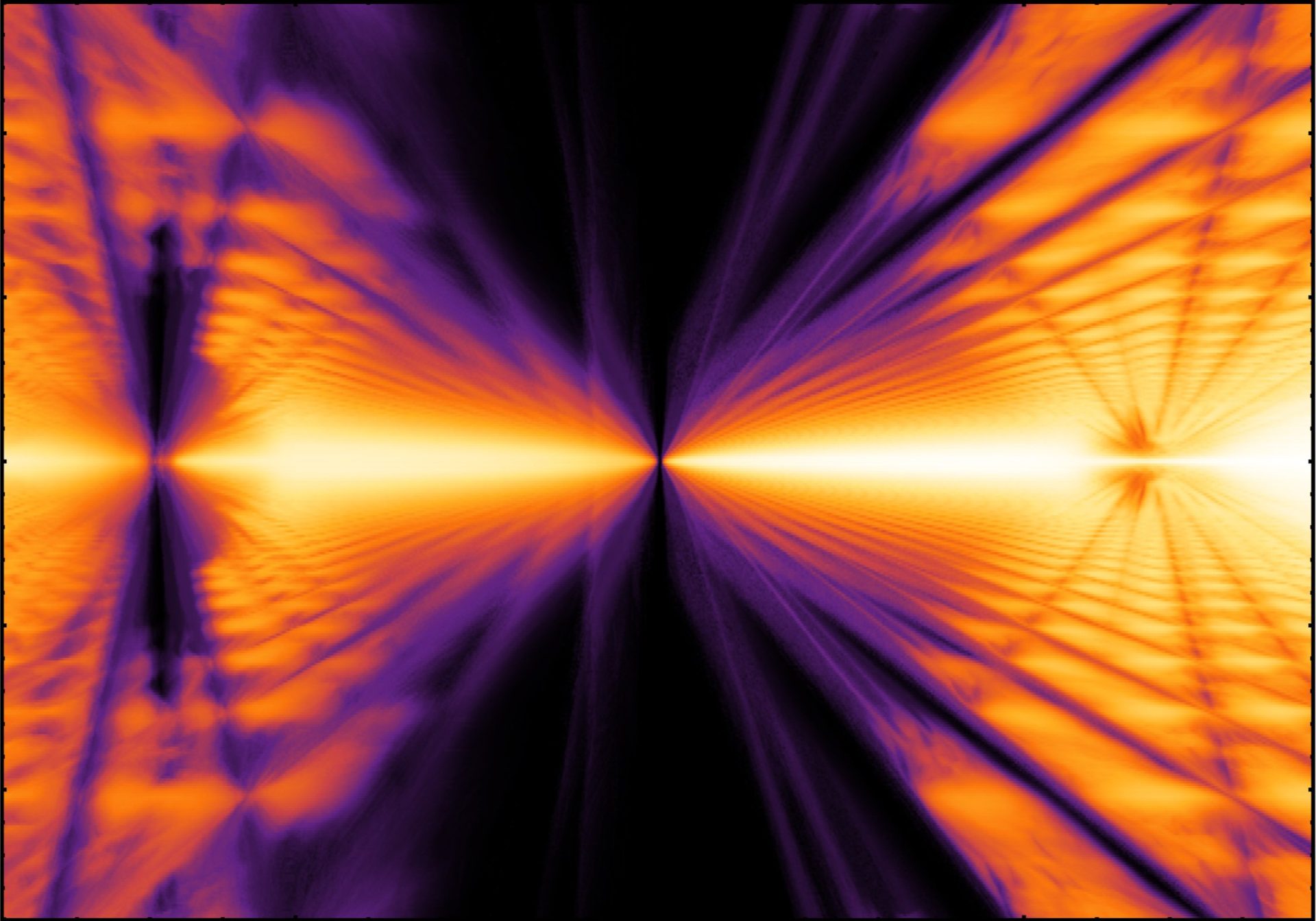
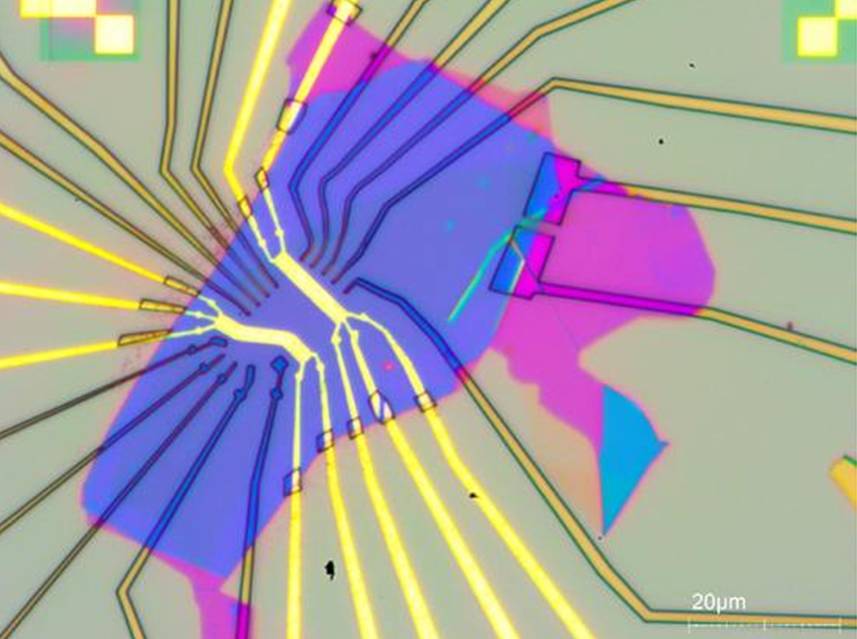
Elecron quantum optics in graphene
Quantum computing is based on the manipulation of quantum bits (qubits) to enhance the efficiency of information processing. In solid-state systems, two approaches have been explored:
- static qubits, coupled to quantum buses used for manipulation and information transmission,
- flying qubits which are mobile qubits propagating in quantum circuits for further manipulation.
Flying qubits research led to the recent emergence of the field of electron quantum optics, where electrons play the role of photons in quantum optic like experiments. This has recently led to the development of electronic quantum interferometry as well as single electron sources. As of yet, such experiments have only been successfully implemented in semi-conductor heterostructures cooled at extremely low temperatures. Realizing electron quantum optics experiments in graphene, an inexpensive material showing a high degree of quantum coherence even at moderately low temperatures, would be a strong evidence that quantum computing in graphene is within reach.
One of the most elementary building blocks necessary to perform electron quantum optics experiments is the electron beam splitter, which is the electronic analog of a beam splitter for light. However, the usual scheme for electron beam splitters in semi-conductor heterostructures is not available in graphene because of its gapless band structure. I propose a breakthrough in this direction where pn junction plays the role of electron beam splitter.
Contact:
Circuit quantum electrodynamics
Owing to the probabilistic character of discrete charge transfers through a quantum conductor, such as quantum point contacts or Josephson junctions, a dc bias produces quantum current fluctuations which couple to the electromagnetic environment of the conductor. Our group investigates the resulting dynamics in its many facets: The emitted microwave radiation naturally conveys information on charge transport in ns timescales otherwise hardly accessible. Moreover, being emitted by a quantum source, this radiation displays quantum correlations as well. Not the least, the electrodynamic coupling can be engineered in order to provide a strong measurement backaction on the transport properties of the conductor itself, where the equivalent of the fine structure constant is of order 1. Such regime is totally unparallled by usual quantum electrodynamics. Beyond aiming at providing a unified quantum description of electrical transport and electromagnetic radiation, this activity brings the oportunity to develop new quantum devices.
Contact: Carles ALTIMIRAS
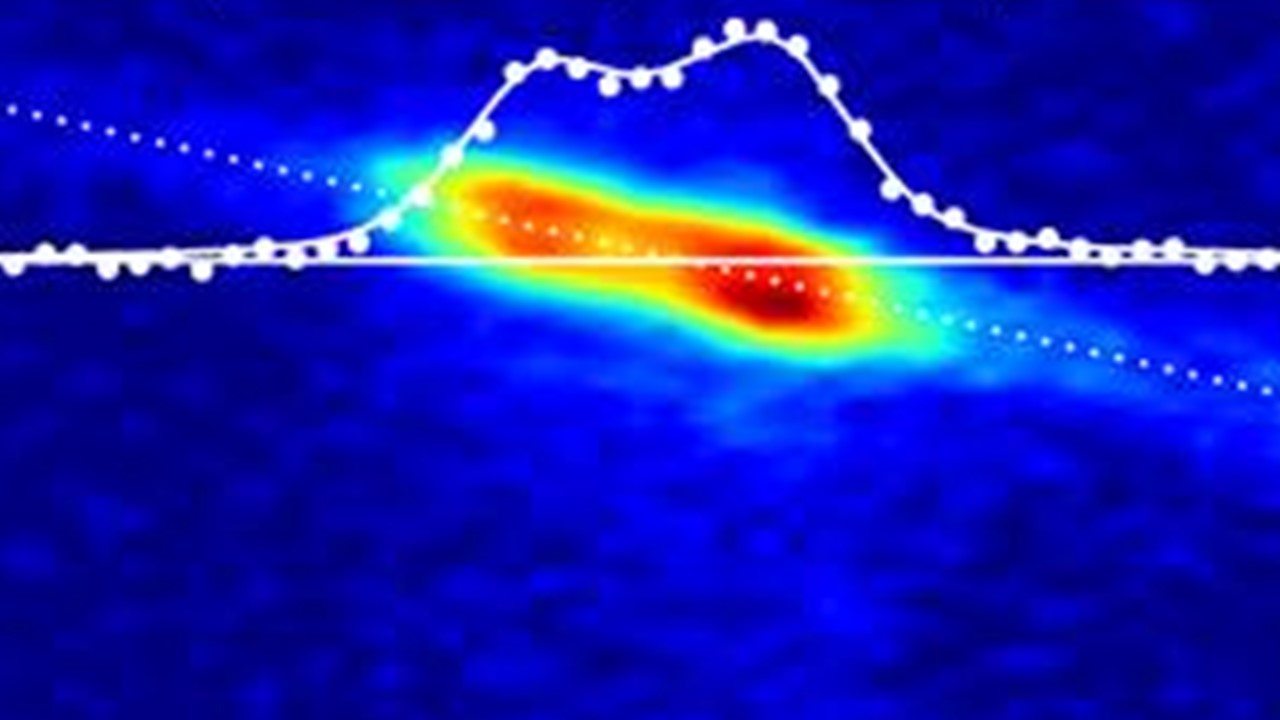
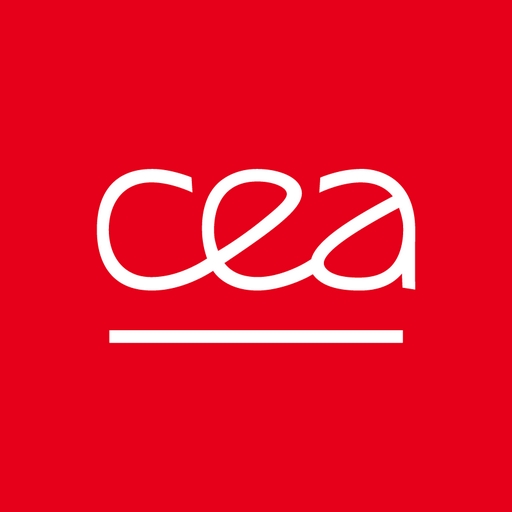
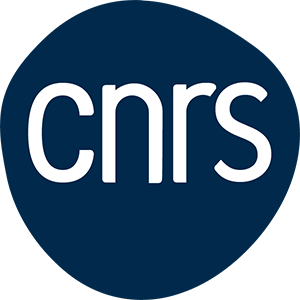
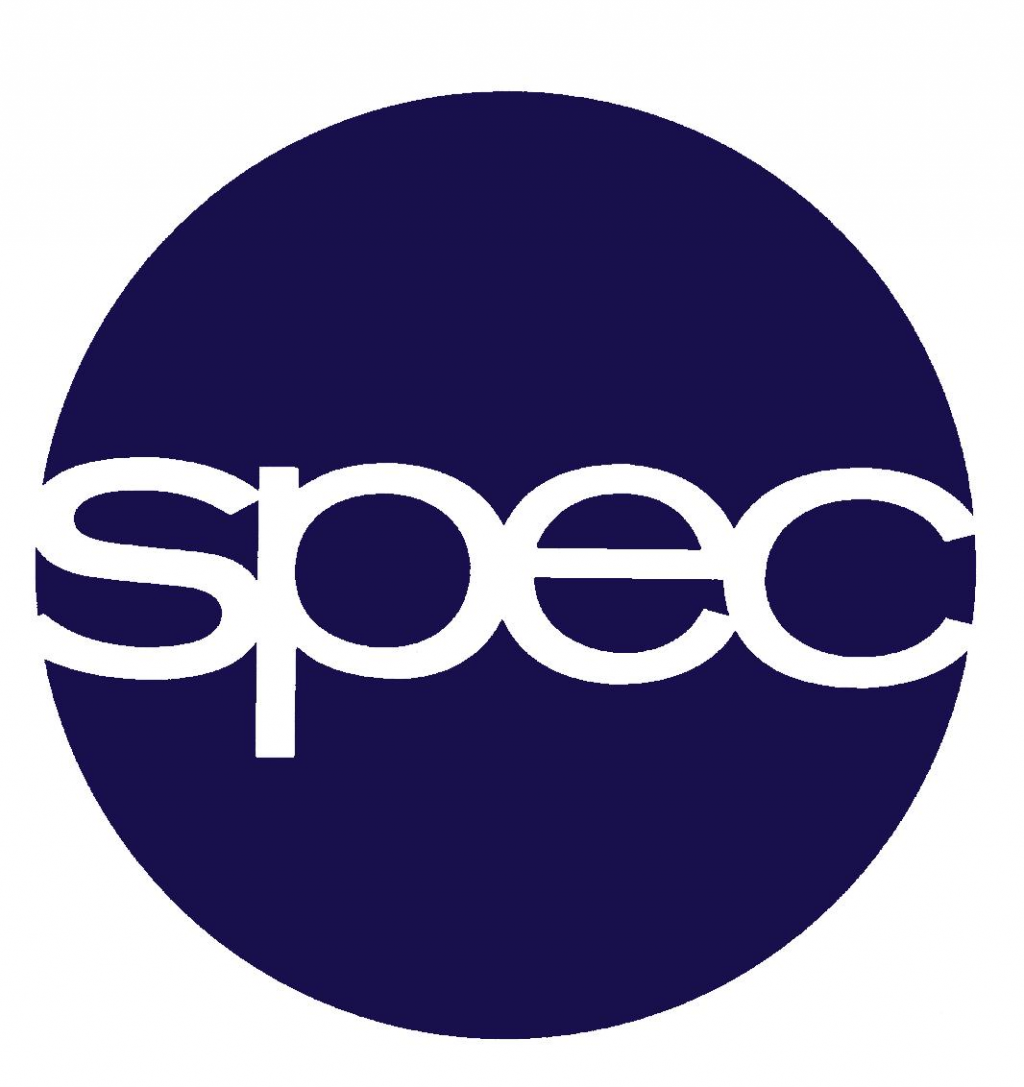
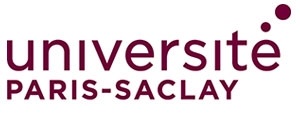
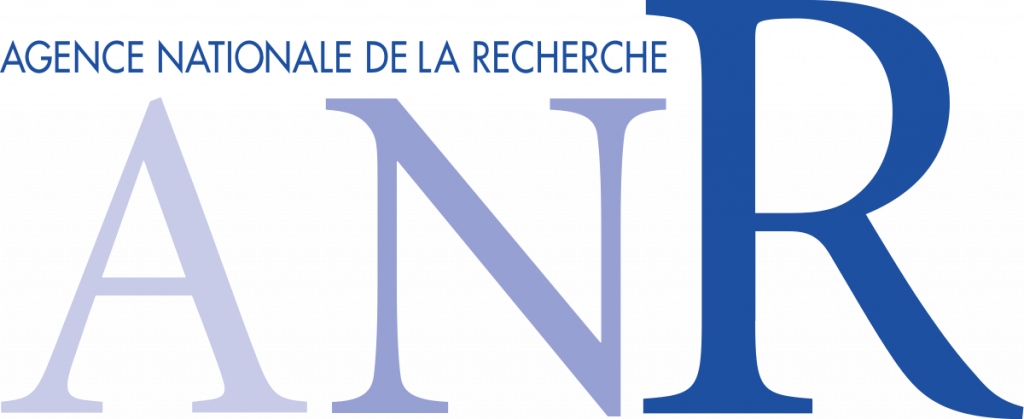
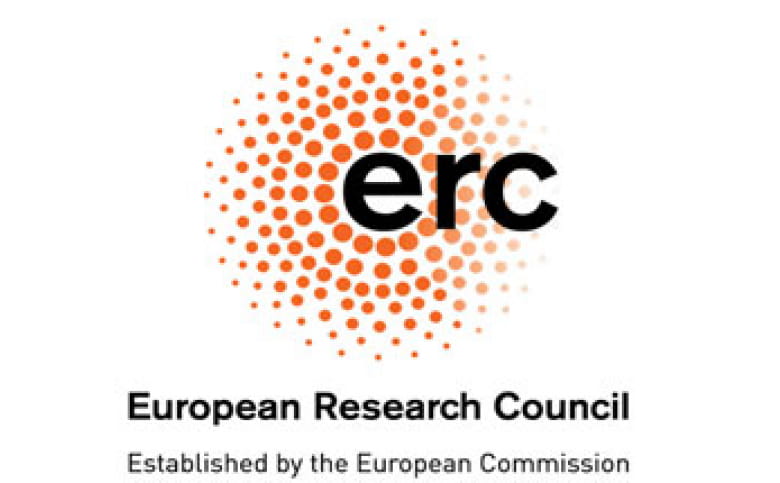