Graphene is a gapless semiconductor, meaning that it always conducts electricity, even at low temperature. However, under high magnetic field, it becomes an insulator, and its nature is still far from being fully understood. Researchers of SPEC, in collaboration with teams of the C2N (Palaiseau, France) and NIMS (Tsukuba, Japan) have probed the thermal transport properties of this peculiar insulating state at low temperature and high magnetic field. They found that it is also a thermal insulator, in contradiction with recent theoretical predictions.
Under high magnetic field and at low temperature, graphene exhibits an unusual insulating state called ν=0, which stems from the interplay between electronic interactions and the intrinsic spin and valley symmetries of graphene. Despite almost two decades of intense theoretical and experimental work, the nature of ν=0 still remains a highly active and fascinating research domain. In particular, the various candidate ground states of ν=0 are quantum Hall ferromagnets with different spin and valley polarizations, providing a rich phase diagram with several experimentally accessible knobs. While most experiments probing ν=0 to date are based on electron transport, several recent theoretical works have suggested turning to thermal transport.
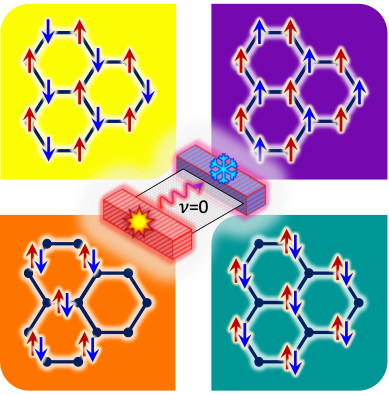
The four possible ground states of ν=0, shown as two spins (red and blue arrows) distributed on the honeycomb lattice of graphene. Yellow: antiferromagnetic phase: the two opposite spins reside on a separate sublattice. Purple: ferromagnetic phase: the two spins are aligned, each on its sublattice. Orange: Kekule distorsion phase: the two opposite spins live on a superposition of the two sublattices. Cyan: sublattice polarized phase: the two opposite spins live on the same sublattice. The central cartoon depicts the principle of the experiment, where heat is carried for a hot electrode (red) to a cold one (purple) across ν=0. Only the antiferromagnetic and Kekule distorsion phase are thermal conductors at low temperature.
Indeed, not only is it quite evident to use thermal transport to probe an electrically insulating system, but, more fundamentally, the ferromagnetic nature of ν=0 endows its candidate ground states with a low energy excitation spectrum involving collective spin and valley waves which do not carry charge, but carry heat. Thus, the low temperature thermal transport properties of ν=0 directly reflect its ground state; particularly, the two most likely candidates have gapless spin/valley waves spectra, yielding a finite thermal conductance even at very low temperature.
To probe the thermal conductance of ν=0, we have used a technique implemented at SPEC to measure thermal transport in graphene in the quantum Hall effect regime. It relies on the fact that Joule power is only dissipated in the electrodes of a sample in the quantum Hall regime, allowing to define hot electron reservoirs which exchange heat across a ν=0 region of a monolayer graphene sample. We have adapted this technique to several samples in complementary geometries allowing to probe both charge and thermal transport at ν=0.
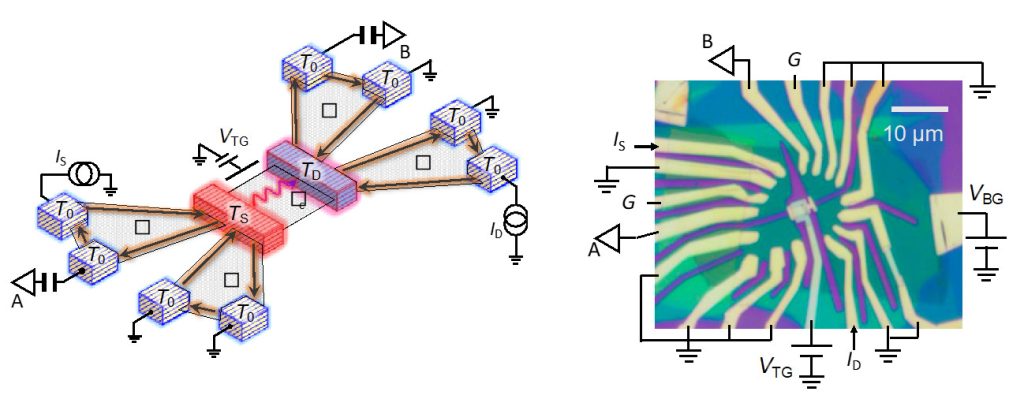
Left: sketch of the “two-terminal” thermal transport geometry, allowing to measure the thermal flow between the source (red brick) and detector (purple brick) electrodes. The external parts of the sample allow controlling a measuring the source and detector temperature TS and TD. Right: optical micrograph of one of the devices fabricated to implement this geometry, along with the schematized experimental wiring.
Surprisingly, our experiment showed that no matter the sample geometry, magnetic field, and accessible temperatures, there was no significant thermal flow through ν=0, suggesting that it is both an electrical and thermal insulator. While this would point to one of the two thermally insulating ground states, the inconsistencies with previous experiments and the theoretical predictions hint that none of the candidate ground states may actually be thermal conductors, thereby calling for further experimental and theoretical investigations.
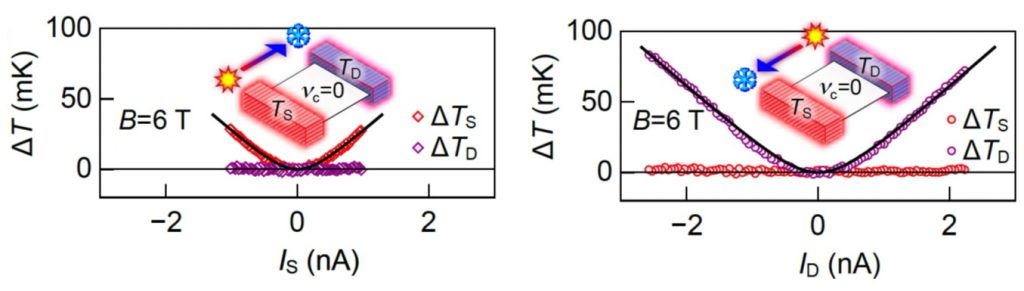
Typical results of the experiment. Left: temperatures of the source (red) and detector (purple) versus source heating current. As the source heats up, the detector stays at low temperature, heralding an absence of heating. Right: reverse situation with the detector being heated up, also leading to an absence of heating in the source.
Our results are published simultaneously with those obtained by a team at IISC Bengaluru (India), also demonstrating demonstrating a negligible heat flow for ν =0 in bilayer graphene, where the same physics is at play.
Reference:
Vanishing bulk heat flow in the ν = 0 quantum Hall ferromagnet in monolayer graphene
R. Delagrange, M. Garg, G. Le Breton, A. Zhang, Q. Dong, Y. Jin, K. Watanabe, T. Taniguchi, P. Roulleau, O. Maillet, P. Roche, and F. D. Parmentier, Nature Physics, in press (2024)
doi: 10.1038/s41567-024-02672-0