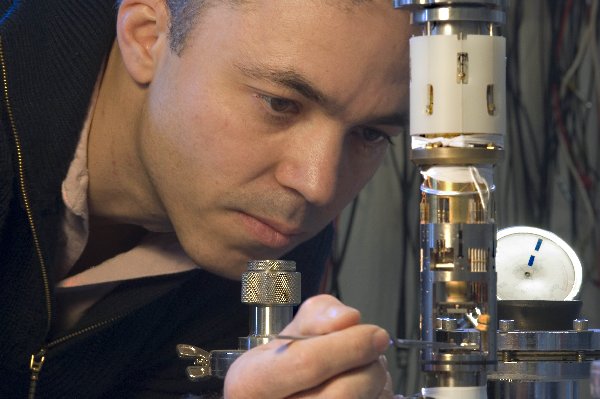
Position
- Senior researcher
Research fields
– Multiferroics :
The coupling of magnetic, electric and mechanical properties within a unique material is a most looked after characteristic that only very few compounds possess. These so-called ‘multiferroics’ have seen a recent surge of interest as magneto-electric (ME) effects, i.e. the coupling between electrical polarization and magnetism, has a great potential for making memories applied in future information technologies. This property is indeed of great interest in order to write magnetic memories (used e.g. in Magnetic Random Access Memories, MRAMs), efficiently and without significant heat production. The search for an ideal material has revived the investigation of known compounds, of which BiFeO3 stands out as the most important example as it is still the only one with ferroelectricity and antiferromagnetism coexisting at room temperature. Although it has been known for a long time, it remains a subject of intensive investigations aiming to improve and understand its properties in bulk and thin film forms.
In our group, we have chosen to study BiFeO3 in detail. Our work started in 2006 with the success in growing, in our laboratory, exceptional quality single crystals [1] which are magnetically and electrically single domain. This allowed us to measure its intrinsic polarisation at room temperature in excess of 100 mC/cm2 [1], a record among all ferroelectrics. Using neutron scattering on similar crystals, we evidenced for the first time in bulk BiFeO3 a strong coupling between magnetic and ferroelectric orders [2] (figure). We then demonstrated the magnetic exchange between the antiferromagnetic lattice of BiFeO3 crystals and a soft ferromagnetic layer deposited on top, which could also be manipulated with the polarisation state of the multiferroic to demonstrate an electric control of the magnetic anisotropy [3, 4]. In 2010, we also discovered that BiFeO3 is photostrictive, i.e. it changes size when illuminated by visible light [4]. This is a very interesting property which can be used to couple photons to phonons or realize new types of memories [5] using remnant photostrictive states. Moreover, due to its not too high bandgap, BiFeO3 is also a photovoltaic material in which photovoltages and photoconductance can be controlled by an electric field [6]. Despite the reduced PV efficiency, this last property is potentially interesting, as is the exact (still unclear) role played by its ferroelectric domain walls. We designed and carried out an original experiment allowing to image photovoltages around a unique domain wall in a single crystal [7] evidencing the importance of internal fields.
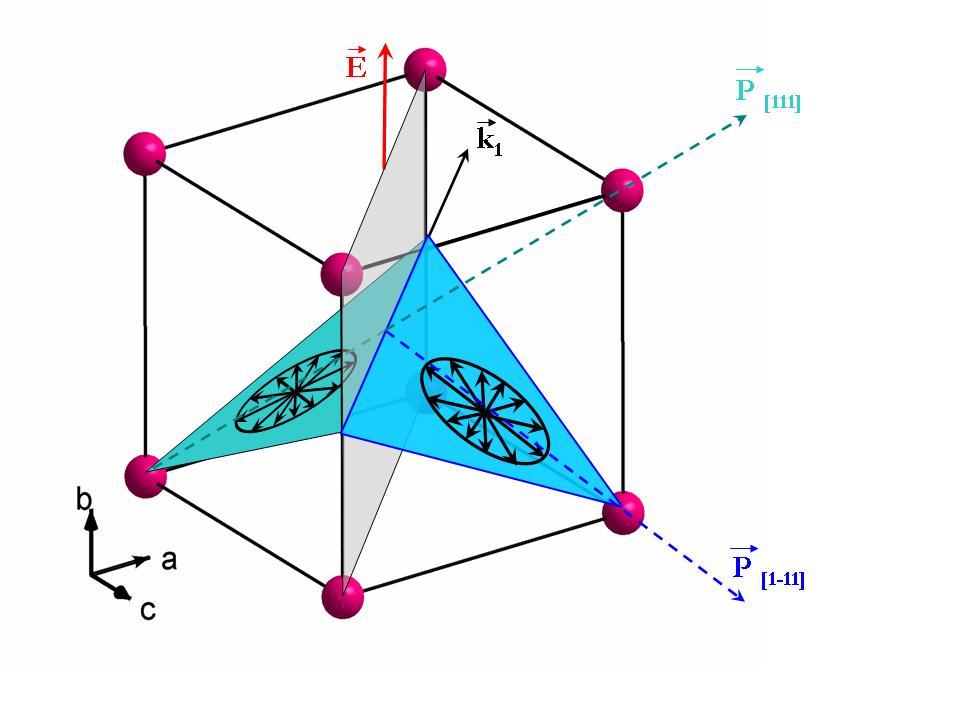
Figure: locking between ferroelectric polarization and magnetic order (antiferromagnetic cycloid)
References:
1. Very large spontaneous electric polarization in BiFeO3 single crystals at room temperature and its evolution under cycling fields, D. Lebeugle, D. Colson, A. Forget, and M. Viret, Appl. Phys. Lett. 91, 022907 (2007).
2. Electric-field-induced spin-flop in BiFeO3 single crystals at room-temperature, D. Lebeugle, D. Colson, A.Forget, M. Viret, A. M. Bataille and A. Gukasov, Phys. Rev. Lett. 100, 227602 (2008).
3. Electric Field Switching of the Magnetic Anisotropy of a Ferromagnetic Layer Exchange Coupled to the Multiferroic Compound BiFeO3, D. Lebeugle, A. Mougin, M. Viret, D. Colson, and L. Ranno, Phys. Rev. Lett. 103, 257601 (2009).
4. Coupling between an incommensurate antiferromagnetic structure and a soft ferromagnet in the archetype multiferroic BiFeO3/cobalt system, M. Elzo, R. Moubah, C. Blouzon, M. Sacchi, S. Grenier, R. Belkhou, S. Dhesi, D. Colson, F. Torres, M. Kiwi, M. Viret, N. Jaouen, Phys. Rev. B 91, 014402 (2015).
5. Optical Writing of Magnetic Properties by Remanent Photostriction, V. Iurchuk, D. Schick, J. Bran, D. Colson, A. Forget, D. Halley, A. Koc, M. Reinhardt, C. Kwamen, N. A. Morley, M. Bargheer, M. Viret, R. Gumeniuk, G. Schmerber, B. Doudin, and B. Kundys, Phys. Rev. Lett. 117, 107403 (2016).
6. Photoelectric effects in single domain BiFeO3 crystals, Reda Moubah, Olivier Rousseau, Dorothée Colson, Alla Artemenko, Mario Maglione, and Michel Viret, Advanced Functional Materials 22, 4814 (2012).
7. Photovoltaic response around a unique 180 degrees ferroelectric domain wall in single-crystalline BiFeO3, C. Blouzon, J.-Y. Chauleau, A. Mougin, S. Fusil and M. Viret, Phys. Rev. B 94, 094107 (2016).
– Antiferromagnetic spintronics :
Among the ordered electronic states occurring in materials, magnetism is uniquely robust and ferromagnets have proven very important in everyday life. They also compose the elemental bricks of ‘spintronics’, the new branch of electronics using the electron spin in devices for information technology. Despite their success, these materials have fundamental physical limitations, which might be circumvented by using antiferromagnets (AF), compounds with counter-aligned neighbouring magnetic moments. These have several key potential advantages including insensitivity to external perturbations like electromagnetic fields and a reduced mutual interaction with their neighbouring domains, which could lead to the production of denser memories in the near future. In our group, we have chosen to study insulating antiferromagnets because of their superior dynamical properties. Indeed, antiferromagnets have intrinsic dynamics in the THz range thus providing the fantastic opportunity to multiply by a hundred the speed of operating magnetic devices. However, this potential revolution comes at some price, as it is very hard to control antiferromagnetic orders.
In the last years, we have worked on the two fronts of spin conduction in insulators and antiferromagnetic imaging. The former started a few years ago with our first demonstration that insulating antiferromagnets are spin conductors [8]. This is an important conceptual discovery as we realized that a spin current can flow without conduction electrons. We proposed that the conducting entities are spin excitations in the form of intrinsically spin charged antiferromagnetic waves, or antiferromagnons. Several theories have appeared since this result but this point is still not yet settled.
We also addressed very recently another important issue related to imaging AF textures. This is a much harder task than for ferromagnets (because of the absence of stray fields) and we have developed a non-linear optics technique based on imaging the second harmonic light generated by magnetic orders. The experiment based on femtosecond light pulses has state of the art submicron resolution and it allowed us to follow the evolution of AF domains under the application of some external fields [9]. This provides an essential tool for pursuing investigations on how to best control AF domains and eventually to trigger and use antiferromagnetic spin waves in THz spintronic devices. In parallel, we have been able to image the uncompensated moments from the non-collinear cycloidal antiferromagnetic structures in BiFeO3 in a collaboration with the UMR CNRS/Thales and the university of Montpellier [10]. This demonstrates that NV center magnetometry is suitable for imaging domain walls in pure collinear antiferromagnets.
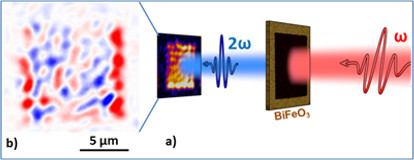
Figure: Principle of second harmonic generation imaging. b) Reconstructed image showing the AF domains in G type BiFeO3 on SrTiO3.
References:
8. Conduction of spin currents through insulating antiferromagnetic oxides, C. Hahn, G. de Loubens, V. V. Naletov, J. Ben Youssef, O. Klein and M. Viret, Europhys. Lett. 108, 57005 (2014).
9. Multi-stimuli manipulation of antiferromagnetic domains assessed by second harmonic imaging, J.-Y. Chauleau, E. Haltz, C. Carrétéro, S. Fusil and M. Viret, Nature Materials 16, 803 (2017).
10. Real-space imaging of non-collinear antiferromagnetic order with a single spin magnetometer, I. Gross, W. Akhtar, V. Garcia, L. J. Martinez, S. Chouaieb, K. Garcia, C. Carrétéro, A. Barthélémy, P. Appel, P. Maletinsky, J.-V. Kim, J. Y. Chauleau, N. Jaouen, M. Viret, M. Bibes, S. Fusil and V. Jacques, Nature 549, 252 (2017).
– Spin-orbit based spintronics :
Spintronics relies on the discrimination of spin up and down carriers originally generated by charge currents in metallic ferromagnets. Recent developments aim to get rid of this source of dissipation by manipulating pure spin currents without the charge counterpart. One convenient way of producing pure spincurrents is to use the spin-orbit coupling (SOC) interaction in a non-magnetic heavy metal like Pt. The interaction, called Spin Hall Effect (SHE), relies on a directional scattering of electrons of different spins by SOC resulting in the generation of a spin current transverse to a charge current. As signals should eventually be read as voltages, the reverse effect, called inverse Spin Hall effect, converts a spin current into a transverse charge current. Another SOC effect based on the Rashba interaction is even more efficient. The effect stems from the joint action of the SOC and built-in electric potentials in two-dimensional electron gases existing at surfaces, interfaces or semiconductor quantum wells. We have measured this so-called Inverse (Rashba) Edelstein Effect (IEE) in the Ag/Bi interface [11], where the Rasha splitting is particularly strong. In the experiments, spin currents are generated by spin pumping using ferromagnetic resonance (FMR) in an adjacent ferromagnet (figure). We have also demonstrated that other systems like the 2D electron liquid appearing at the interface between two insulators as in the LaAlO3/SrTiO3 are also excellent candidates [12]. Some properties linked to this particular system are still not understood. In particular, we have shown that there is an in-plane dependence of the effect that probably reflects the non-isotropic Fermi surface inducing either an angular dependence of the Rashba field or a direction dependent scattering time. This could be linked to the symmetry of the relevant in-plane d-orbitals, but this is not yet clear. Moreover, time-resolved measurements would be highly desirable in order to elucidate carrier lifetime effects, but this is a hard task as present measurements rely on spin pumping by ferromagnetic resonance, a technique intrinsically limited to the nanosecond range.
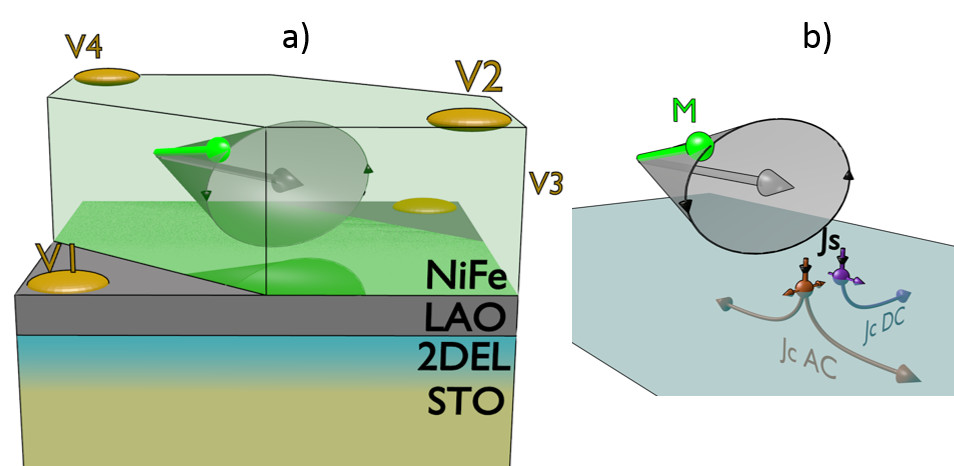
Figure: Spin injection by ferromagnetic resonance (spin pumping) from NiFe into the electron liquid at the LAO/STO interface
References:
11. Control of the spin to charge conversion using the inverse Rashba-Edelstein effect, S. Sangiao, J. M. De Teresa, L. Morellon, I. Lucas, M. C. Martinez-Velarte, M. Viret, Appl. Phys. Lett. 106, 172403 (2015).
12. Efficient spin-to-charge conversion in the 2D electron liquid at the LAO/STO interface, J.-Y. Chauleau, M. Boselli, S. Gariglio, R. Weil, G. de Loubens, J.-M. Triscone, M. Viret, Europhys. Lett. 116, 17006 (2016).
– Nanoscale magnetism:
In the last few years, we have studied the interaction between magnetisation dynamics and electrical transport in magnetic nanostructures and atomic contacts. We have achieved atomic sized resolution in the detection of ferromagnetic resonance using a novel experimental setup in which a mechanical break junction experiment is set up in a radio-frequency compatible environment where electrical measurements can be carried out under variable static and dynamical magnetic fields. The resonance properties of magnetic nanostructures were first measured in order to determine the reliability and the high sensitivity of our system. Then the susceptibility of domain walls in nanostuctures at frequencies higher than those classically used for their motion, was measured and found to be large and almost independent of the frequency [13]. This susceptibility was roughly 10 times that obtained in saturated domains. The experimental setup also allowed the study of the interaction between spin currents and the magnetization dynamics of nanostructures at the ferromagnetic resonance. The spin currents dynamically generated at the FMR have been measured in Py/Pt nanostructures using the inverse spin Hall effect in platinum [14]. The influence on the FMR of spin current injection using the spin Hall effect in Pt has also been observed.
The FMR of atomic contacts has also been studied. While breaking our nanostructures of cobalt and permalloy, new resonant modes have been shown to appear at fields higher than that of the uniform resonance. This is attributed to the effect of demagnetization fields that are locally modified when reducing the constriction diameter. In the atomic contact regime, we have measured the constrained domain wall resonance. In that case the rectified signal, generated by a few atoms, can reach values 1000 times larger than those generated by the FMR of saturated domains, before controllably breaking the constrictions [15].
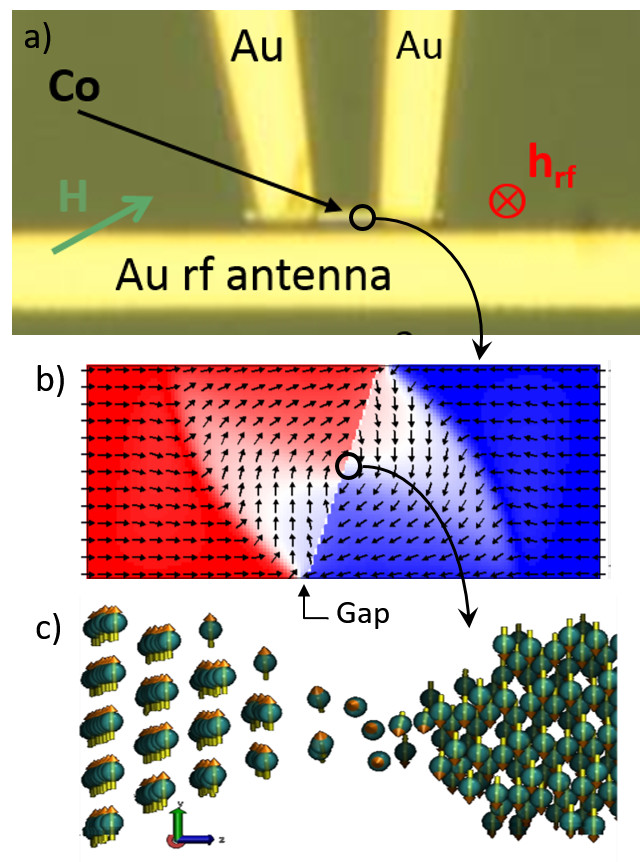
Figure: Co break junction with RF antena. A domain wall is positioned on the atomic contact and a DC voltage appears across the contact at the resonance frequency.
13. Large RF susceptibility of transverse domain walls, O Rousseau, S Petit-Watelot and M Viret, J. Phys.: Condens. Matter 24, 024211 (2012).
14. Interaction between ferromagnetic resonance and spin currents in nanostructures, O. Rousseau and M. Viret, Phys. Rev. B 85 (2012) 144413
15. Giant rectification from magnetization dynamics of an atomically sharp domain wall, O. Rousseau, D. Beaujouan, S. Petit-Watelot, P. Thibaudeau, S. Rohart and M. Viret, submitted.
CV
Academic record :
· 2003: Habilitation à diriger les recherches (Paris VI). Subject: “Effets résistifs liés aux variations locales de l’aimantation dans les matériaux ferromagnétiques”
· 1993/96: Post-doctoral fellowship in Trinity College, Dublin (Ireland). Recipient of an individual ‘Marie Curie’ grant.
· 1992 : European doctorate from the Université P. et M. Curie (Paris VI). Subject: «Elaboration de couches minces supraconductrices BiSrCaCuO et étude de leurs propriétés de transport».
· 1990: Master of Science by research from Trinity College, Dublin. Subject: “Transport properties of copper oxide superconducting thin films”.
· 1988-89: Scientific attaché of the French embassy in Ireland as part of my ‘National Service’ duties.
· 1987: science degree from the ‘Ecole Nationale Supérieure de Physique de Grenoble (INPG/ENSPG)’ and post-graduate ‘DEA’ from ‘Université Joseph Fourrier, Grenoble.
Other Publications (selection)
Publications within the HAL-CEA basis.
Oxides:
Electron localization in mixed-valence manganites, J.M.D. Coey, M. Viret, L. Ranno and K. Ounadjela, Phys. Rev. Lett. 75, 3910 (1995).
Magnetic localization in mixed-valence manganites, M. Viret, L. Ranno and J.M.D. Coey, Phys. Rev. B 55, 8067 (1997).
Low-field colossal magnetoresistance in manganite tunnel spin valves, M. Viret, M. Drouet, J.P. Contour, J. Nassar, C. Fermon and A. Fert, Europhys. Lett. 39, 545 (1997).
Magnetic coherence above the Curie point in ferromagnetic manganites, M. Viret, H. Glattli, C. Fermon, A.M. de Leon-Guevara and A. Revcolevschi, Europhys. Lett. 42, 301 (1998).
Colossal resistive relaxation effects in a Pr0.67Ca0.33MnO3 single crystal, A. Anane, J.P. Renard, L. Reversat, C. Dupas, P. Veillet, M. Viret, L. Pinsard and A. Revcolevschi, Phys. Rev. B 59, 256 (1999).
Mixed-valence manganites, J.M.D. Coey, M. Viret and S. von Molnár, Advances in Physics 48, 167 – 292 (1999).
Spin polarised tunnelling as a probe of half metallic ferromagnetism in mixed-valence manganites, M. Viret, J. Nassar, M. Drouet, J.P. Contour; C. Fermon and A. Fert, J. Magn. Magn. Mat. 198-199, 1 (1999).
Interface magnetism of La0.7Sr0.3MnO3 thin films studied by neutron reflectometry, F. Ott, M. Viret, R. Borges, R. Lyonnet, E. Jacquet, C. Fermon and J.P. Contour, J. Magn. Magn. Mat. 211, 200-205 (2000).
A CrO2-based magnetic tunnel junction, A. Barry, J.M.D. Coey and M. Viret, J. Phys. Cond. Mat. 12, L173 (2000).
Magnetic filaments in resistive manganites, M. Viret, F. Ott, J.P. Renard, H. Glattli, L. Pinsard, A. Revcolevschi, Phys. Rev. Lett. 93, 217402 (2004).
Magnetotransport properties of Fe3O4 epitaxial thin films: Thickness effects driven by antiphase boundaries, A.V. Ramos, J.B. Moussy, M.J. Guittet, A.M. Bataille, M. Gautier-Soyer, M. Viret, C. Gatel, P. Bayle-Guillemaud, E. Snoeck, J. Appl. Phys. 100, 103902 (2006).
Very large spontaneous electric polarization in BiFeO3 single crystals at room temperature and its evolution under cycling fields, D. Lebeugle, D. Colson, A. Forget, and M. Viret, Appl. Phys. Lett. 91, 022907 (2007).
Room-temperature coexistence of large electric polarization and magnetic order in BiFeO3 single crystals, D. Lebeugle, D. Colson, A. Forget, M. Viret, P. Bonville, J. F. Marucco, and S. Fusil, Phys. Rev. B 76, 024116 (2007).
Magnetoresistance in NiOx nanoconstrictions controlled by magnetic fields and currents, O. Cespedes, M. Viret and J.M.D. Coey, J. Appl. Phys. 103, 083901 (2008).
Electric-field-induced spin-flop in BiFeO3 single crystals at room-temperature, D. Lebeugle, D. Colson, A.Forget, M. Viret, A. M. Bataille and A. Gukasov, Phys. Rev. Lett. 100, 227602 (2008).
Artificial antiphase boundary at the interface of ferrimagnetic spinel bilayers, A.V. Ramos, S. Matzen, J.B. Moussy, F. Ott, M. Viret, Phys. Rev. B 79, 014401 (2009).
Mixed-valence manganites (Reprinted from Advances in Physics, vol 48, pg 167-293), J.M.D. Coey, M. Viret and S. von Molnar, Advances in Physics 58, 571 (2009) and Mixed-valence manganites – ten years on, Advances in Physics 58, 567 (2009).
Electric Field Switching of the Magnetic Anisotropy of a Ferromagnetic Layer Exchange Coupled to the Multiferroic Compound BiFeO3, D. Lebeugle, A. Mougin, M. Viret, D. Colson, and L. Ranno, Phys. Rev. Lett. 103, 257601 (2009).
Exchange coupling with the multiferroic compound BiFeO3 in antiferromagnetic multidomain films and single-domain crystals, D. Lebeugle, A. Mougin, M. Viret, D. Colson, J. Allibe, H. Bea, E. Jacquet, C. Deranlot, M. Bibes, M. and A. Barthelemy, Phys. Rev. B 81, 134411 (2010).
Neutron diffraction study of the BiFeO3 spin cycloid at low temperature, J. Herrero-Albillos, G. Catalan, J.A. Rodriguez-Velamazan, M. Viret, D. Colson, J.F. Scott, J. Phys.: Condens. Matter 22, 256001 (2010).
Light-induced size changes in BiFeO3 crystals, B. Kundys, M. Viret, D. Colson and D. O. Kundys, Nature Materials 9, 803 (2010).
Direct imaging of both ferroelectric and antiferromagnetic domains in multiferroic BiFeO3 single crystal using X-ray photoemission electron microscopy, R. Moubah, M. Elzo, S. El Moussaoui, D. Colson, N. Jaouen, R. Belkhou and M. Viret, Appl. Phys. Lett. 100, 042406 (2012).
beta-NaFeO2, a new room-temperature multiferroic material, M. Viret, D. Rubi, D. Colson, D. Lebeugle, A. Forget, P. Bonville, G. Dhalenne, R. Saint-Martin, G. André and F. Ott, Materials Research Bulletin 47, 2294-8 (2012).
Electronic transitions in strained SmNiO3 thin films, S. Catalano, M. Gibert, V. Bisogni, O.E. Peil, F. He, R. Sutarto, M. Viret, P. Zubko, R. Scherwitzl, A. Georges, G. A. Sawatzky, T. Schmitt and J.-M Triscone, APL Materials 2, 116110 (2014).
Tailoring the electronic transitions of NdNiO3 films through (111)(pc) oriented interfaces, S. Catalano, M. Gibert, V. Bisogni, F. He, R. Sutarto, M. Viret, P. Zubko, R. Scherwitzl, G. A. Sawatzky, T. Schmitt, J.-M. Triscone, APL Mater. 3, 062506 (2015).
Interfacial Control of Magnetic Properties at LaMnO3/LaNiO3 Interfaces, M. Gibert, M. Viret, A. Torres-Pardo, C. Piamonteze, P. Zubko, N. Jaouen, J.-M. Tonnerre, A. Mougin, J. Fowlie, S. Catalano, A. Gloter, O. Stephan, J.-M. Triscone, Nano Lett. 15, 7355 (2015).
Interlayer coupling through a dimensionality-induced magnetic state, M. Gibert, M. Viret, P. Zubko, N. Jaouen, J.-M. Tonnerre, A. Torres-Pardo, S. Catalano, A. Gloter, O. Stéphan & J.-M. Triscone, Nat. Comm. 7, 11227 (2016).
Spintronics:
Spin-scattering in ferromagnetic thin films, M. Viret, D. Vignoles, D. Cole, J. M. D. Coey, W. Allen, D. Daniel, J. E. Gregg, Phys. Rev. B 53, 8464 (1996).
Giant magnetoresistance effects in a single element magnetic thin film, J.F. Gregg, W. Allen, K. Ounadjela, M. Viret, M. Henn, S.M. Thompson and J.M.D. Coey, Phys. Rev. Lett. 77(8), 1580 (1996).
Anisotropy of domain wall resistance, M. Viret, Y. Samson, P. Warin, A. Marty, F. Ott, E. Sondergard, O. Klein and C. Fermon, Phys. Rev. Lett. 85, 3962 (2000).
Individual domain wall resistance in submicron ferromagnetic structures, R. Danneau, P. Warin, J.P. Attane, I. Petej, C. Beigne, C. Fermon, O. Klein, A. Marty, F. Ott, Y. Samson and M. Viret, Phys. Rev. Lett. 88, 157201 (2002).
Electron-magnon scattering and magnetic resistivity in 3d ferromagnets, B. Raquet , M. Viret, E. Sondergard, O. Cespedes, R. Mamy, Phys. Rev. B 66, 024433 (2002).
Magnetoresistance through a single nickel atom, M. Viret, S. Berger, M. Gabureac, F. Ott, D. Olligs, I. Petej, J.F. Gregg, C. Fermon, G. Francinet, G. Le Goff, Phys. Rev. B 66, 220401 (2002).
Electronic noise in magnetic low-dimensional materials and nanostructures, B. Raquet, M. Viret, M. Costes, M. Baibich, M. Pannetier, M. Blanco-Mantecon, H. Rakoto, A. Maignan, S. Lambert and C. Fermon, J. Magn. Magn. Mat. 258, 119 (2003).
Current induced distortion of a magnetic domain wall, X. Waintal and M. Viret, Europhys. Lett. 65, 427 (2004).
Magneto-resistance in nanocontacts induced by magnetostrictive effects, M. Gabureac, F. Ott, C. Fermon, M. Viret, Phys. Rev. B 69, 100401 (2004).
Magnetoresistance of mechanically stable Co nanoconstrictions, M.I. Montero, R.K. Dumas, G. Liu, M. Viret, O.M. Stoll, W.A.A. Macedo and Ivan K. Schuller, Phys. Rev. B 70, 184418 (2004).
Current induced pressure on a tilted magnetic domain wall, M. Viret, A. Vanhaverbeke, F. Ott, J.-F. Jacquinot, Phys. Rev. B 72 (14), 140403 (2005).
Bidomain state in exchange biased FeF2/Ni, O. Petracic, Zhi-Pan Li, Igor V. Roshchin, M. Viret, R. Morales, X. Batlle, and Ivan K. Schuller, Appl. Phys. Lett. 87 (22), 222509 (2005).
Giant Anisotropic Magneto-Resistance in ferromagnetic atomic contacts, M. Viret M. Gabureac, F. Ott, C. Fermon, C. Barreteau, G. Autes, and R. Guirado-Lopez, Eur. Phys. J. B 51, 1 (2006).
Simple model of current induced spin torque in domain walls, A. Vanhaverbeke and M. Viret, Phys. Rev. B 75, 024411 (2007).
Ballistic magnetoresistance? B. Doudin and M. Viret, J. Phys.: Condens. Matter 20, 083201 (2008).
Giant orbital moments are responsible for the anisotropic magnetoresistance of atomic contacts, G. Autes, C. Barreteau, M.C. Desjonqueres, D. Spanjaard and M. Viret, Europhys. Lett. 83, 17010 (2008).
Nonlocal properties of a multidomain magnetic configuration, A. Vanhaverbeke, O. Klein, M. Viret, and J. Ben Youssef, Phys. Rev. B 80, 184414 (2009).
Positive domain wall resistance in atomic-sized constrictions, A. Ben Hamida, O Rousseau, S Petit-Watelot and M Viret, Europhys. Lett. 94, 27002 (2011).
Large RF susceptibility of transverse domain walls, O Rousseau, S Petit-Watelot and M Viret, J. Phys.: Condens. Matter 24, 024211 (2012).
Comparative measurements of inverse spin Hall effects and magnetoresistance in YIG/Pt and YIG/Ta, C. Hahn, G. de Loubens, O. Klein, M. Viret, V. V. Naletov, J. Ben Youssef, Phys. Rev. B 87, 174417 (2013).
Detection of Microwave Spin Pumping Using the Inverse Spin Hall Effect, C. Hahn, G. de Loubens, M. Viret, O. Klein, V. V. Naletov, and J. Ben Youssef, Phys. Rev. Lett. 111, 217204 (2013).
Electrical detection of internal dynamical properties of domain walls, Soraya Sangiao, Michel Viret, Phys. Rev. B 89, 104412 (2014).
Full Control of the Spin-Wave Damping in a Magnetic Insulator Using Spin-Orbit Torque, A. Hamadeh, O. D. Kelly, C. Hahn, H. Meley, R. Bernard, A. H. Molpeceres, V. V. Naletov, M. Viret, A. Anane, V. Cros, S. O. Demokritov, J. L. Prieto, M. Munoz, G. de Loubens, and O. Klein, Phys. Rev. Lett. 113, 197203 (2014).
Unexpected Magnetic Properties of Gas-Stabilized Platinum Nanostructures, O. Cespedes, M. Wheeler, T. Moorsom, M. Viret, M, Nano Letters 15, 45 (2015).
Teaching
Lecturer in M2 (Masters level): ‘magnetism and spin electronics’ in ‘Materials for Energy’ of the INSTN master course.
Projects, grants
Various
Supervisor of the following PhD works:
Mihai Gabureac : ‘Spin polarized electrical transport in ferromagnetic atomic constrictions’, Paris VI, 2004
Antoine Vanhaverbeke : ‘Correlations entre heterogeneites magnetiques et transport electrique dans des nanostructures’, Paris VI, 2006
Delphine Lebeugle : ‘Etude de la coexistence du magnétisme et de la ferroélectricité dans les composés multiferroïques BiFeO3 et Bi0.45Dy0.55FeO3‘, Paris XI, 2007
Aymen Ben Hamida : ‘Transport électrique et transfert de spin dans des constrictions de tailles nanométriques’, Paris VI, 2010
Olivier Rousseau : ‘Effets electriques lors de la resonance ferromagnetique de nanostructures et de contacts atomiques’, Paris VI, 2011
Camille Blouzon : ‘Etude des propriétés photoélectriques et magnétiques des parois de domaines multiferroïques’, Paris VI, 2016