In a ferromagnetic material, the mutual interaction between the spins of the electrons tends to align them side by side, forming a macroscopic magnet. Depending on the structure of the material, an antiferromagnetic arrangement is also possible, where adjacent spins are oriented in opposite directions, leading to zero overall magnetization. Depending on the intensity and nature of the interactions between spins, it is also possible to form small local entities, where the orientation of the spins whirls around as in a vortex. This particular topology gives these objects, known as “skyrmions ”*, enhanced stability, enabling them to be used as highly mobile nanometer-sized magnetic bits.
Many advances have been made recently in the study and use of ferromagnetic skyrmions, but “antiferromagnetic skyrmions” seem to have even greater potential for use, as their mobility is predicted to be a hundred times greater.
Here it is shown that it is possible to create such topological objects, whose orientation can be stabilized and manipulated in antiferromagnetic nanostructures with multiferroic properties. This result points the way to a technology that enables the creation of antiferromagnetic objects, the first step towards the potential realization of non-volatile memories of very high density and very low energy consumption.
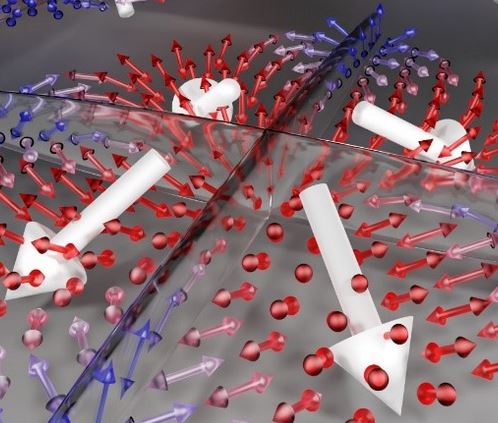
Skyrmion refers to a local vortex orientation of spins. This very particular topology makes them stable on the nanometric scale and at room temperature. They are therefore considered the ultimate magnetic bit for ultra-high-density information storage. This type of entity has been observed and manipulated over the past decade in ferromagnetic materials, where they exhibit intrinsic spin-wave resonance dynamics in the GHz (109 Hz) range. In antiferromagnetic materials, whose electronic structure can be decomposed into two antiparallel spin sublattices, this intrinsic dynamic is 1000 times faster, reaching the THz range (1012 Hz), raising the prospect of ultrafast spintronics. Nevertheless, creating and electrically annihilating antiferromagnetic skyrmions is an incredible challenge, given the low sensitivity of these materials to external magnetic perturbations.
Following an original approach, a collaboration of researchers has found a way to stabilize such topological entities at room temperature and change their orientation on an antiferromagnetic support.
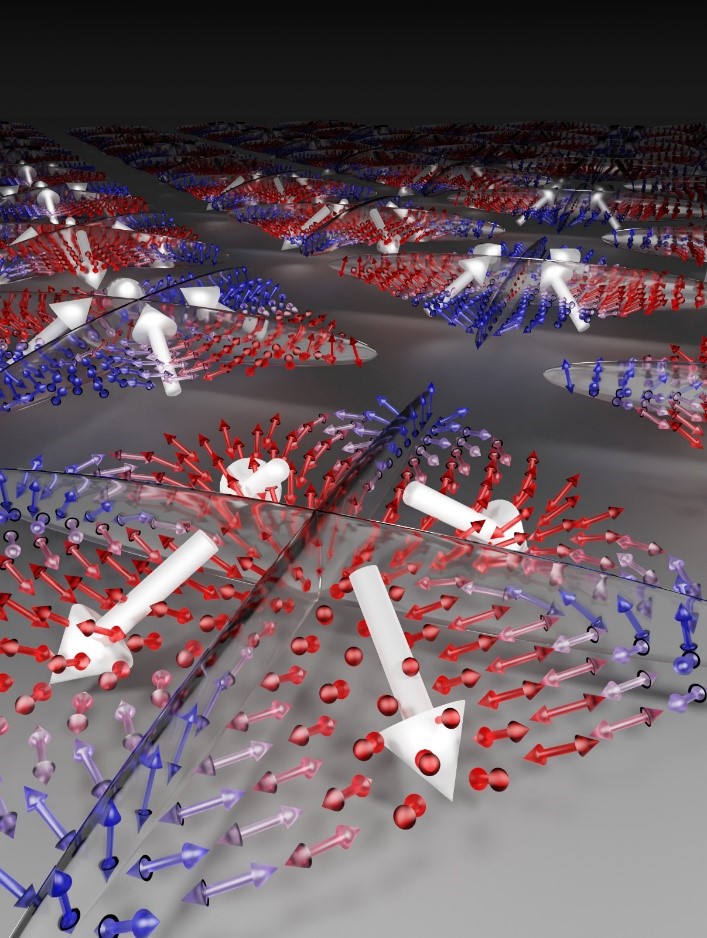
Artistic view of magnetoelectric control of antiferromagnetic spin textures (colored double arrows) by locally converging or diverging ferroelectric domains (white arrows). The framing ferroelectric structure results from local polarization imposed by a voltage pulse applied to the tip of an AFM microscope.
The method uses the coupling between ferroelectric order (associated with electrical charge distributions in the material) and antiferromagnetic order in a very special multiferroic material layer: bismuth ferrite (BiFeO3). This layer exhibits antiferromagnetic order, which replaces the naturally non-collinear spin-cycloid order of the material, due to the stress imposed by growth on a substrate with a different crystalline parameter (DyScO3 or SmScO3). A tiling of adjacent ferroelectric domains (see figure) is then obtained by applying a voltage pulse to the tip of an AFM microscope. Each ferroelectric domain wall intersection then defines a local 4-quadrant structure that can present two possible polarizations, depending on the sign of the applied radial electric field. Coupling with the spins then produces antiferromagnetic textures of distinct orientation, defining two states 0 and 1. It is thus possible to invert the local polarization of the ferroelectric domains from convergent to divergent orientation (see figure) and, by magnetoelectric coupling, the orientation of the antiferromagnetic structure.
Un avantage majeur de cette approche d’encodage réversible et non volatil de l’information à base de matériau antiferromagnétique multiferroïque est qu’il est ainsi possible de basculer entre les deux états magnétiques en appliquant une simple impulsion de tension. Par principe, cette manipulation ultrarapide de l’information entre les deux états est donc très peu consommatrice d’énergie, ce qui pourrait permettre de concevoir des dispositifs de stockage très haute densité et peu consommateurs d’énergie.
This results are published in Nature Materials.
Reference:
“Electric-field induced multiferroic topological solitons”,
Arthur Chaudron, Zixin Li, Aurore Finco, Pavel Marton, Pauline Dufour, Amr Abdelsamie, Johanna Fischer, Sophie Collin, Brahim Dkhil, Jirka Hlinka, Vincent Jacques, Jean-Yves Chauleau, Michel Viret, Karim Bouzehouane, Stéphane Fusil & Vincent Garcia, Nature Materials, (2024)– Publié le 6 mai 2024
See the CNRS-Physique news: “Vers une spintronique ultrarapide et économe en énergie“.
Contact CEA : Michel Viret, SPEC/LNO, UMR SPEC CEA-CNRS, Université Paris-Saclay.
*Collaboration :
- Laboratoire Albert Fert (CNRS / Thales / Université Paris-Saclay)
- SPEC/LNO, Service de Physique de l’Etat Condensé (SPEC, CEA / CNRS / Université Paris-Saclay),
- Laboratoire Charles Coulomb (L2C, CNRS / Université de Montpellier),
- Laboratoire Structure, Propriétés et Modélisation des Solides (SPMS, CNRS / Centralesupélec / Université Paris-Saclay)
- Institute of Physics, Academy of Sciences of the Czech Republic (République Tchèque).