Researchers from the Nanoelectronics and the Modeling and Theory groups of SPEC, in collaboration with experimentalists from NTT-BRL and NIMS (Japan) and theorists from KAIST (Korea) have developed new, fully tunable electronic beam splitters in graphene, that directly rely on the crystalline symmetries of graphene. Using these beam splitters, the researchers have demonstrated the implementation of an electronic Mach-Zehnder interferometer which splits and recombines electronic wavepackets along a p-n junction realized in graphene. The analysis of the observed electronic quantum interferences strongly suggests that graphene could be used to realize complex quantum circuits with a very high degree of robustness to decoherence.
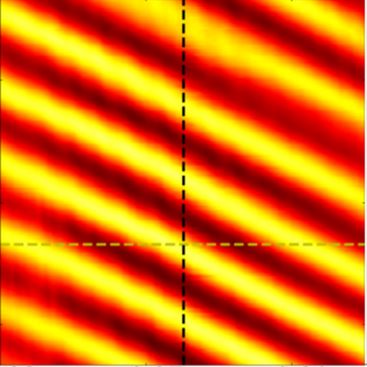
The field of electron quantum optics relies on the analogy between the propagation of electrons in a quantum conductor and that of photons in quantum optics experiments. This research field emerged in the late nineties with the possibility of manipulating electron beams in condensed matter systems while preserving their wave-particle nature. It has proven since then to grant a fundamental understanding of quantum electronics down to the single-particle excitation. The prototypical systems of electron quantum optics are two-dimensional conductors in the quantum Hall effect regime. This regime is reached under strong perpendicular magnetic field and is characterized by the existence of one-dimensional, chiral and dissipationless electronic channels propagating along the edges of the sample. Those (quantum Hall) edge channels can be directly viewed as the analog of optical fibers for electrons. While a large majority of these experiments have been performed in semiconductor heterostructures such as GaAs/GaAlAs, graphene has recently become the subject of intense attention. Indeed, not only does graphene present a rich, new physics in the quantum Hall regime, but it is also thought to offer vastly superior coherence properties, allowing to envision more complex experiments that could lead to the development of quantum information processing schemes based on the control of the quantum trajectories of single charges in a circuit.
However, a key ingredient for electron quantum optics had been lacking in graphene: a tunable electronic beam splitter, allowing to controllably divide and combine electronic wavepackets onto different edge channels while preserving quantum coherence. This is mainly due to the fact that graphene is a semimetal, without bandgap, hindering the development of usual field-effect structure such as the quantum point contacts widely used as electronic beam splitters in GaAs/GaAlAs. Instead of trying to engineer a tunable bandgap in graphene, the researchers from SPEC and their collaborators have relied on the valley pseudospin, a fundamental degree of freedom of graphene intimately linked to its crystalline structure, to control the coupling between edge channels. By taking advantage of the crystalline disorder present at the edges of an otherwise ultra-clean graphene sample, the researchers have demonstrated the mixing of edge channels with opposite valley degree of freedom (around e.g. the top yellow region in Fig.1, see also Fig.2(a)). Crucially, they have also demonstrated the ability to finely tune the mixing by carefully shifting the mixing point along the disordered graphene edge.
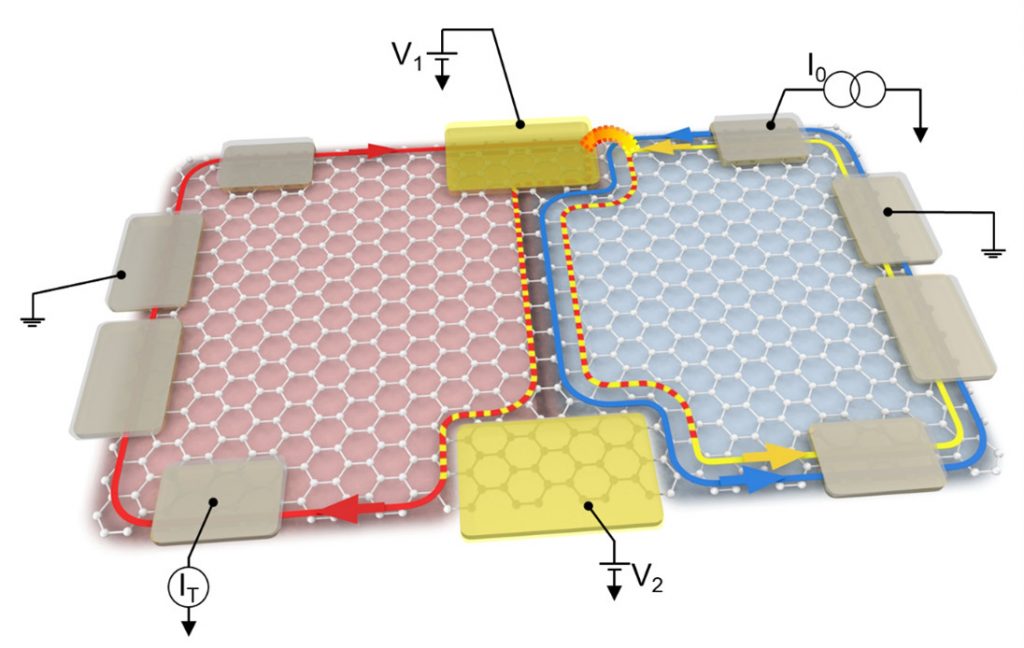
Figure 1 – Artist view of the sample. An ultra-clean graphene flake is divided in two regions with opposite (p- and n-) doping (red and blue). Electrostatic gates (highlighted in yellow) placed on the corners of the interface between the two regions are used to control the mixing between the edge channels (red and yellow arrow), realizing quantum Hall valley splitters. The whole system forms an electronic Mach-Zehnder interferometer : electrons coming from the top (yellow and red) channels are split by the top beam splitter into two (dashed yellow-red) channels propagating along the p-n interface, and finally recombined at the bottom beam splitter. The phase shift between the two paths of the interferometer is controlled by the external magnetic field.
Having demonstrated the tunable mixing, the researchers have shown that it is a quantum coherent process by combining two such quantum Hall valley splitters so as to form an electronic Mach-Zehnder interferometer along a graphene p-n junction. The dependence of the quantum interferences with the tuning of the beam splitters (see Fig.2(b)) and the bias voltage applied to the interferometer not only confirms that it is indeed in a Mach-Zehnder geometry, but also that its robustness to decoherence likely surpasses by far that of electronic interferometers realized in conventional GaAs/GaAlAS heterostructures. While this apparent robustness warrants further investigation, it is very encouraging for future experiments in more complex geometries, such as the controlled entanglement of a pair of single electron emitted independently.
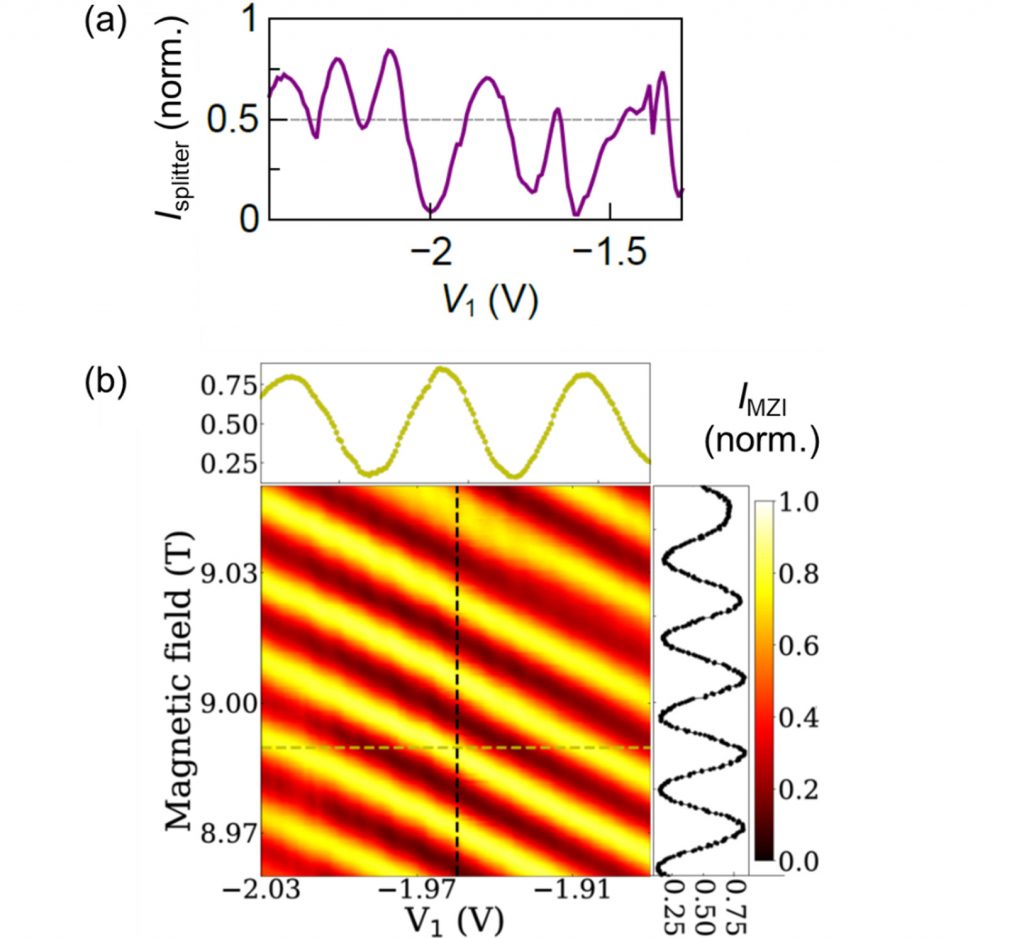
Figure 2 – (a) Electronic current through one of the beam splitters, as a function of its control gate voltage V1. Although irregular, the observed oscillations are stable and reproducible. (b) Electronic current through the Mach-Zehnder interferometer formed by the two beam splitters. Highly regular sine-waves with high contrast are observed as a function of both gate voltage and magnetic field.
This investigation is part of the ERC Starting Grant COHEGRAPH project, and has benefitted from funding from the Labex PALM (Université Paris-Saclay).
Reference :
Quantum Hall valley splitters and a tunable Mach-Zehnder interferometer in graphene, M. Jo, P. Brasseur, A. Assouline, G. Fleury, H. -S. Sim, K. Watanabe, T. Taniguchi, W. Dumnernpanich, P. Roche, D. C. Glattli, N. Kumada, F. D. Parmentier, and P. Roulleau, Phys. Rev. Lett. 126, 146803 (2021) – Editor’s suggestion – Featured in Physics
Contact CEA : François Parmentier and Preden Roulleau, Nanoelectronics Group (SPEC/GNE)
Collaboration :
- Service de Physique de l’Etat Condensé (SPEC), UMR 3680 CEA-CNRS, Université Paris-Saclay
- Department of Physics, Korea Advanced Institute of Science and Technology (KAIST Physics)
- National Institute for Materials Science (NIMS), Japan
- NTT Basic Research Laboratories (NTT-BRL), Japan