2D materials
Since the first extraction of single graphene sheet in 2004, many other two-dimensional (2D) materials have been produced. 2D materials offer an interesting playground for not only revisiting condensed matter but also giving rise to new unexpected phenomena. In GMT many different types of 2D systems are under investigation:
VdW heterostructures: we have focused our research on the electrostatic properties of vdW heterostructures, achieved a p-n junction in graphene, studied the defects in 2D materials and discovered a Dirac cone in blue phosphorene.
Hybrid 2D/molecule systems: this topic ranges from the study of molecular self-assembling on graphene or metallic surface to the modelling and control of molecular doping, reduction, switching, and tautomerization[1] on surface.
Nanostructured graphene & BN twisted bilayers: The effects of nanostructuration (periodic array of “holes” in the honeycomb lattice, twisted bilayer architectures, etc) on the electronic properties of bare 2Ds have been predicted with DFT calculations and dedicated tight-binding (TB) approaches. Deep symmetry/geometry analyses have been performed and optical responses have been extracted
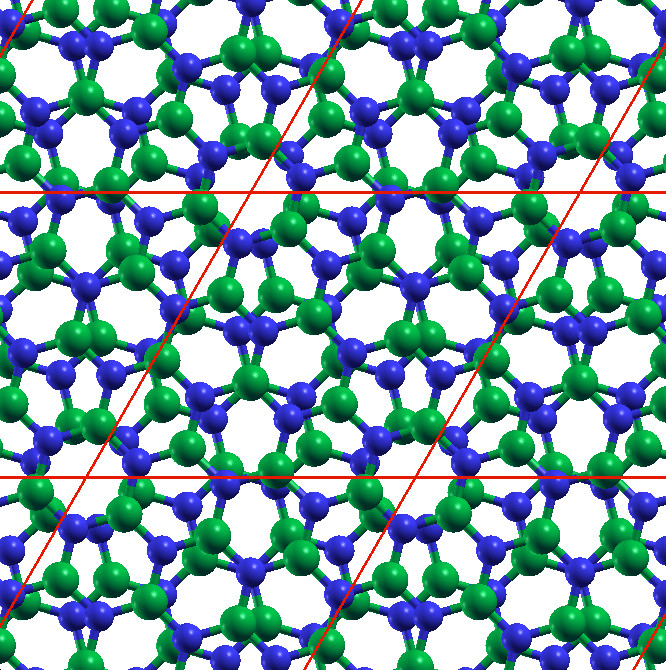
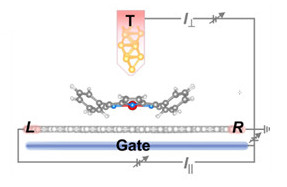
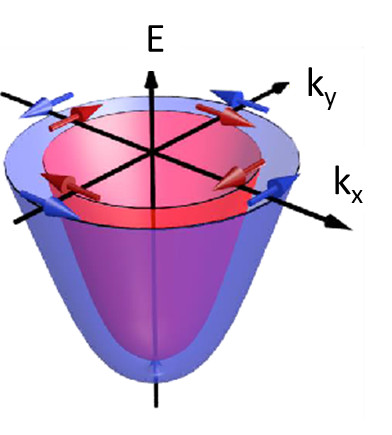
X-tronics
Since the dawn of spin-tronics an avalanche of new X-tronics effects have been proposed often also related to the discovery of new materials (topological insulators, 2D materials, hybrid systems etc.). GMT is involved in various aspects of X-tronics. |
Molecular spintronics: The modelling of various switchable magnetic molecules in interaction with a metallic substrates has been performed in tight-collaboration with experimental partners. Transport in nano & molecular junctions: Electron transport has been explored in a variety of atomic and single-molecule junctions with metallic as well as graphene electrodes. We have determined attenuation factors of the electronic current, and studied the role of orbital symmetry and quantum interferences for perfect spin-filtering effect. Orbitronics: in collaboration with LNO group we have investigated Rashba-Edelstein effect at LAO/STO interface. In particular we showed that orbital response is an order of magnitude larger than spin response and explained the origin of in-plane anisotropy and gate voltage dependence. |
Quantum transport in mesoscopic systems
A system is “mesoscopic” if its size is comparable to the typical phase coherence length of the wavefunctions that it hosts: transport across it is therefore characterized by quantum interference effects:
Time-dependent transport: Electronic systems driven far from equilibrium, in the ultrafast GHz-THz range, offer novel possibilities. We studied e.g. high-harmonic generation from topological surface states
Shaped Dirac matter: Transport in topological insulator nanostructures is determined by the competition between geometry and quantum geometry, and is highly sensitive to magnetic fields.
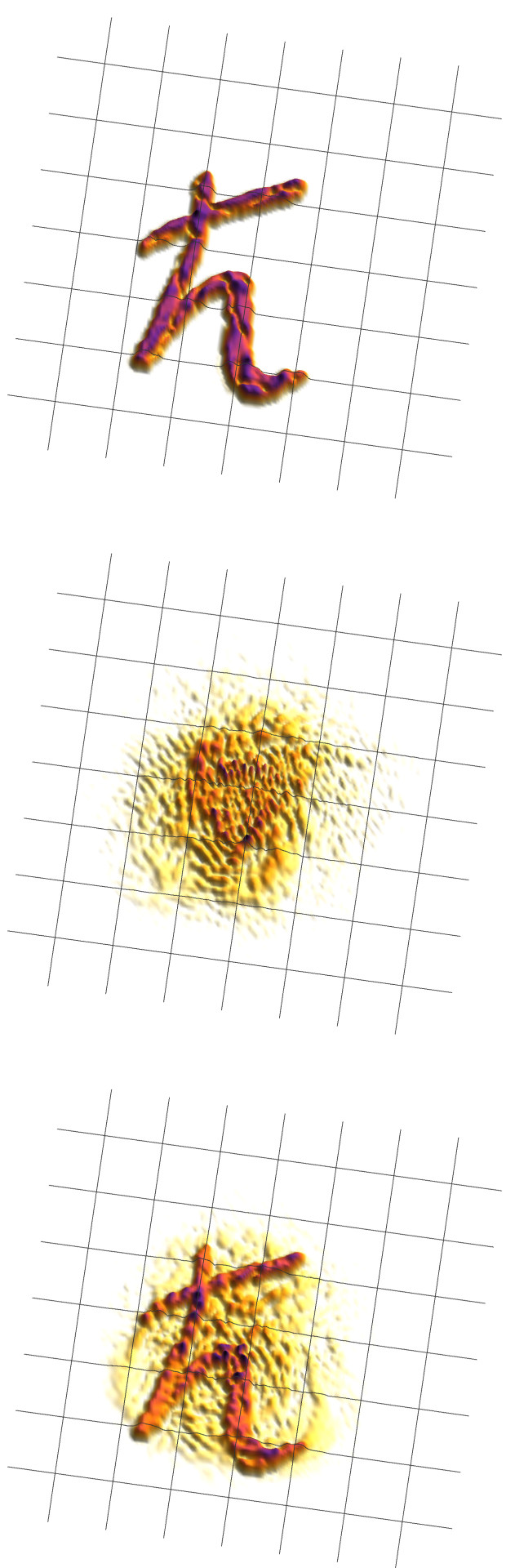
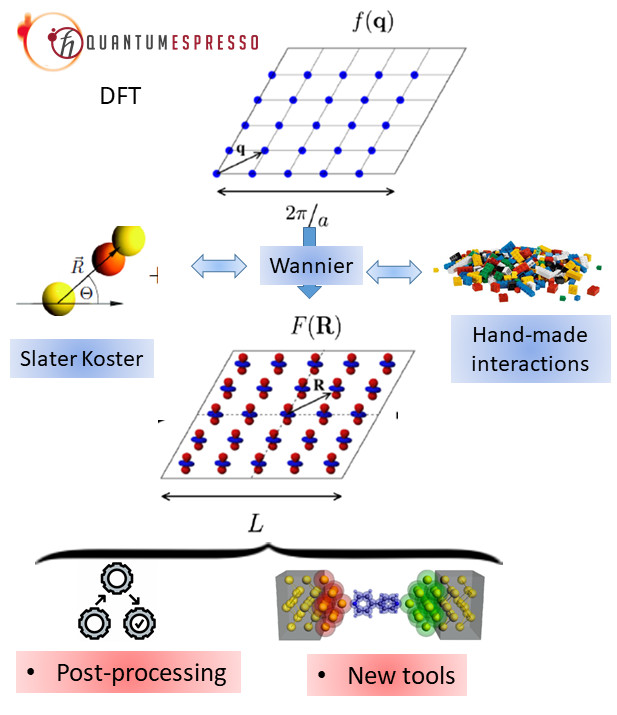
Code development
In the group we have a strong experience in developing modelling approaches based on the TB Hamiltonian at various levels of approximation. We use Wannier functions, to construct a tight-binding model of a real material that captures the essential features at the Fermi surface, as well as more semi-phenomenological approaches fitted DFT or even constructed “by-hand” to include additional interactions. |
A spin dynamics code based on the resolution of the Landau-Lifshitz-Gilbert equation in a non-collinear magnetic tight-binding approach has been developed A time dependent quantum-mechanical methods and codes based on wave packet propagation have been developed for both electron and phonon carriers using Wannier function basis and parameters extracted from ab initio electronic structure calculations using Quantum ESPRESSO (QE) and Wannier90 packages. Different types of interactions, such as electron-phonon, can be included to allow for inelastic effects at the nanocontact |