For energy storage, lithium-oxygen (Li-O2) batteries are seen as a promising alternative to current lithium-ion batteries. They offer much higher theoretical specific energies than current batteries (~ 3500 Wh/kg vs. 160 Wh/kg for Li-ion). However, this technology still poses a number of challenges, the most important being the rapid loss of performance after only a few charge/discharge cycles. The main challenge in developing this technology lies in finding new materials for the positive electrode, often referred to as the “air cathode”.
During operation of the Li-O2 battery, this air cathode is the site of electrochemical reactions involving the reduction of oxygen during discharge or the release of oxygen during charging. The air cathode must also be capable of storing the products formed during discharge, such as lithium peroxide Li2O2, which is insoluble in the aprotic electrolyte (H ion-free) used.
Among the air cathode materials under consideration, porous Metal-Organic Framework (MOF) solids appear to be good candidates. MOFs have a large specific surface area and high porosity. Their open framework structure provides not only a host network for lithium ion diffusion and good oxygen diffusion, but also sufficient space for the deposition of discharge products.
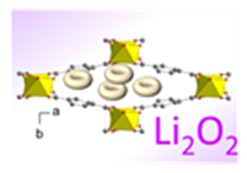
In order to meet future energy storage requirements, new battery technologies with higher capacities need to be developed. Among possible systems, lithium-air/lithium-oxygen technology offers promising prospects. Indeed, their theoretical energy density is significantly higher than that of current lithium-ion batteries (~ 3,500 Wh/kg, i.e. 25% of that of petroleum fuels, vs. 160 Wh/kg for lithium-ion)
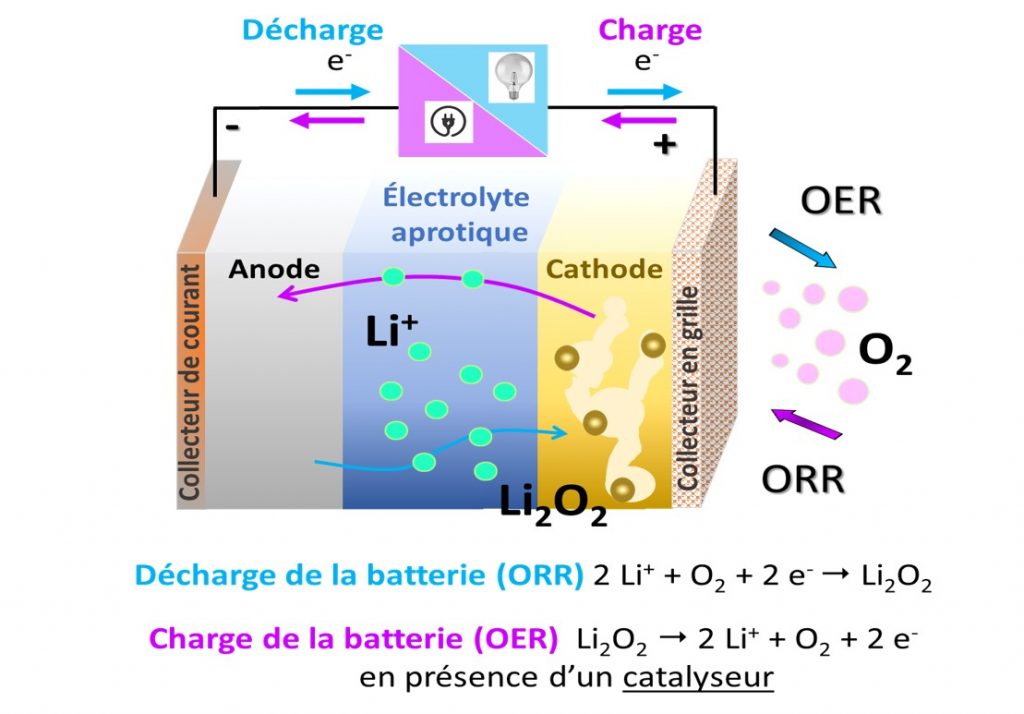
Operating principle of a lithium-oxygen battery in an aprotic organic medium (no H ion): during charging, oxygen reduction (ORR: Oxygen Reduction Reaction) takes place at the porous cathode, while discharge products in the form of Li2O2) decompose during charging (OER: Oxygen Evolution Reaction).
A Li-O2 battery consists of a lithium metal anode and an air cathode separated by a membrane soaked in electrolyte, which may be aprotic, i.e. free of H ions, such as a lithium salt dissolved in an organic solvent, or aqueous (see figure). The air cathode is generally made of porous carbon with a high specific surface area. During discharge, the lithium metal anode oxidizes, releasing Li ions into the electrolyte; the reaction is reversed during charging. In the air cathode, oxygen enters the porous electrode, dissolves in the electrolyte and is reduced (O2 → 2O2-) at the electrode surface during discharge. In an aprotic environment, the O2- ion then reacts with Li ions in the electrolyte to form Li2O2 lithium peroxides. These discharge products are insoluble in organic electrolytes and need to be stored in the porous cathode. During charging, it has been shown that these discharge products can be reversibly decomposed in the presence of a catalyst
Porous hybrid materials of the Metal-Organic Framework (MOF) type are the subject of numerous studies due to their structural diversity and potential applications as energy storage materials
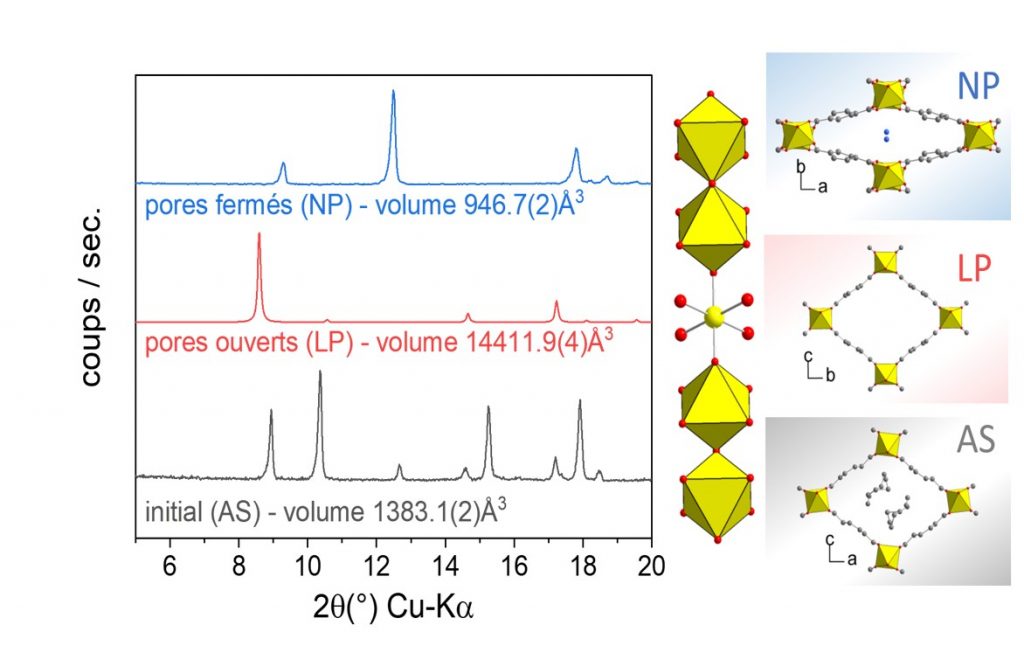
X-ray diffraction patterns (Kα-Cu = 1.54 Å) highlighting the phase transitions observed in the presence of an external stimulus: temperature transition (AS ⇔ LP) or adsorption of water molecules (LP ⇔ NP).
The LEEL/NIMBE team, in collaboration with a team from IMAP at ESPCI, has chosen to study the electrochemical properties of a flexible MOF as an air cathode for batteries
Li-O2
Phase transitions are observed on removal of the synthesis residue or in the presence of water molecules in the pores (see figure below).
Electrochemical tests carried out with MIL-53 electrodes show good performance whatever the synthesis method. As shown in the figure, several behaviors are observed depending on the initial value of the Q1 capacity following the first charge:
- for Q1>900 mAh/g): subsequent capacities drop rapidly and become very low (Q2: 100-300 mAh/g).
- for moderate Q1 values (500-900 mAh/g), the capacity at charge is equivalent to the capacity at discharge, showing that the ORR reaction is taking place.
- for very low Q1 values (< 300 mAh/g): Q2 capacity is high, and the oxygen reduction (ORR) and Li2O2 decomposition (OER) reactions are taking place.
However, whatever the electrochemical behavior, the number of charge/discharge cycles at full capacity remains very limited.
Ex situ studies (DRX, SEM and XPS) were carried out on the dismantled cell after 1 then 10 discharges. These confirm the nature of the discharge product formed (Li2O2) and show that it is well encapsulated in the pores of the MIL-53 solid.
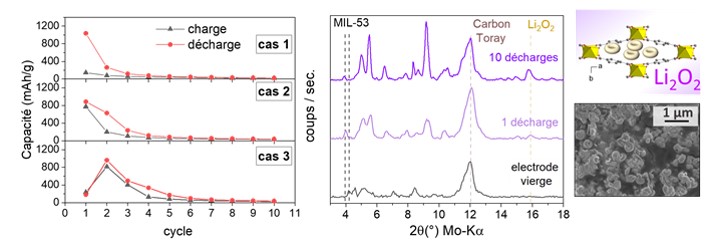
Left: evolution of charge and discharge capacities during the first charge-discharge cycles with MIL-53 electrodes. Right: evolution of X-ray diffraction patterns (Kα-Mo = 0.71 Å) as a function of the number of charge/discharge cycles.
This study shows that flexible MOF-type solids are good candidates as porous cathodes for Li-O2 batteries. However, the low conductivity of these materials and the inertia of the metal center (Al) limit their performance. This work is being pursued by considering other metal centers (Fe, V)
* MIL: Matériau de l’Institut Lavoisier
References:
W.-J. Kwak, Rosy, D. Sharon, C. Xia, H. Kim, L. Johnson, P. Bruce, L. Nazar, Y. Sun, A. Frimer, M. Noked, S. Freunberger, D. Aurbach, Chemical Reviews 120,6626 (2020).
Z. Ma, X. Yuan, L. Li, Z.-F. Ma, D.P. Wilkinson, L. Zhang, J. Zhang, Energy Environ. Sci. 8, 2144 (2015).
X. Zhang, A. Chen, M. Zhong, Z. Zhang, X. Zhang, Z. Zhou, X.-H. Bu, Electrochem. Energ. Rev. 2, 29 (2019).
J. Lee, O. K. Farha, J. Roberts, K. A. Scheidt, S. T. Nguyen, J. T. Hupp, Chemical Society Reviews. 38, 1450 (2009).
Y. Zhang, B. Gikonyo, H. Khodja, M. Gauthier, E. Foy, B. Goetz, C. Serre, S. Coste Leconte, V. Pimenta, S. Surblé, Materials. 14, 4618 (2021).
CEA-IRAMIS contact: Suzy Surblé (NIMBE/LEEL UMR CEA-CNRS).
Collaboration:
- B. Goetz, V. Pimenta & C. Serre
Institut des Matériaux Poreux de Paris (IMAP), ESPCI Paris, Ecole Normale Supérieure de Paris, CNRS, PSL University, 75005 Paris, France - S. Coste Leconte
INSTN, Specialization School for Low-Carbon Energies and Health Technologies, Saclay Teaching Unit, CEA, 91191 Gif-sur-Yvette, France.