Under a high magnetic field and at low temperatures, electrons in graphene can end up with all their spins perfectly aligned. The elementary excitations of such an ideal magnet, called spin waves or magnons, are intrinsically magnetic objects that nonetheless have an electrostatic nature, as they carry an electric dipole.
Researchers from the Nanoelectronics group of SPEC, in collaboration with theorists from IPhT and experimentalists from NTT-BRL and NIMS (Japan), have recently observed for the first time the electric dipole of spin waves in quantum Hall ferromagnet by using an electronic Mach-Zehnder interferometer, realized in a high quality graphene sample, as an ultra-sensitive quantum sensor.
When a perpendicular magnetic field is applied on a two-dimensional electron system like e.g. graphene, electrons become distributed in energy on widely-spaced, highly degenerate Landau levels. The latter are the hallmark of the so-called quantum Hall effect regime. At large enough magnetic field, the various symmetries underlying the Landau levels (spin, valley, etc) can break, giving rise to well-separated sub-Landau levels that can be fully polarized in a given symmetry. By tuning the electron density and the magnetic field such that only one spin-polarized sub-Landau level is filled, one can effectively create a perfect ferromagnet, a system where absolutely all electron spins point in the same direction. In monolayer graphene, such a perfect “quantum Hall ferromagnet” is obtained at filling factor ν=1, when spin and valley symmetries are broken by electronic interactions and only a single spin- and valley-polarized electron channel can propagate along the edges of the sample (while the bulk remains insulating). Recent experiments have shown that it is possible to excite the elementary bulk excitations of this peculiar ferromagnet, which are charge-neutral spin waves, or magnons, simply by applying to the sample a drain-source potential larger than the Zeeman energy. Many fundamental properties of these magnons remain to be observed and investigated. In particular, even though they are magnetic excitations, magnons of a quantum Hall ferromagnet have an intrinsic excitonic nature, as they carry an intrinsic electric dipole oriented perpendicularly to their propagation direction.
To observe this electric dipole, the researchers from SPEC and their collaborators have devised an experiment, described in Figure 1a, in which a stream of magnons is emitted towards an electronic Mach-Zehnder interferometer realized in a graphene p-n junction, acting as an ultra-sensitive electric dipole detector. The detection mechanism is two-fold:
- on the one hand, the stream of magnons impinge on the interferometer with a given average angle, which depends on the position of the magnon emission point relative to the interferometer. Depending on the value of this angle, the positive part of the electric dipole might be closer to the interferometer than the negative part, or vice-versa (see insets in Figure 1a). This plays the role of an effective local electrostatic gate, shifting the position of the interferometer’s two arms (central blue and yellow arrows in Figure 1a) relative to one another, thus leading to a measurable phase shift of the interferometer.
- On the other hand, the magnons are emitted randomly in time, and impinge accordingly on the interferometer. This leads to rapid fluctuations in the electric field felt by the interferometer, which cause the amplitude of the interference pattern to diminish.
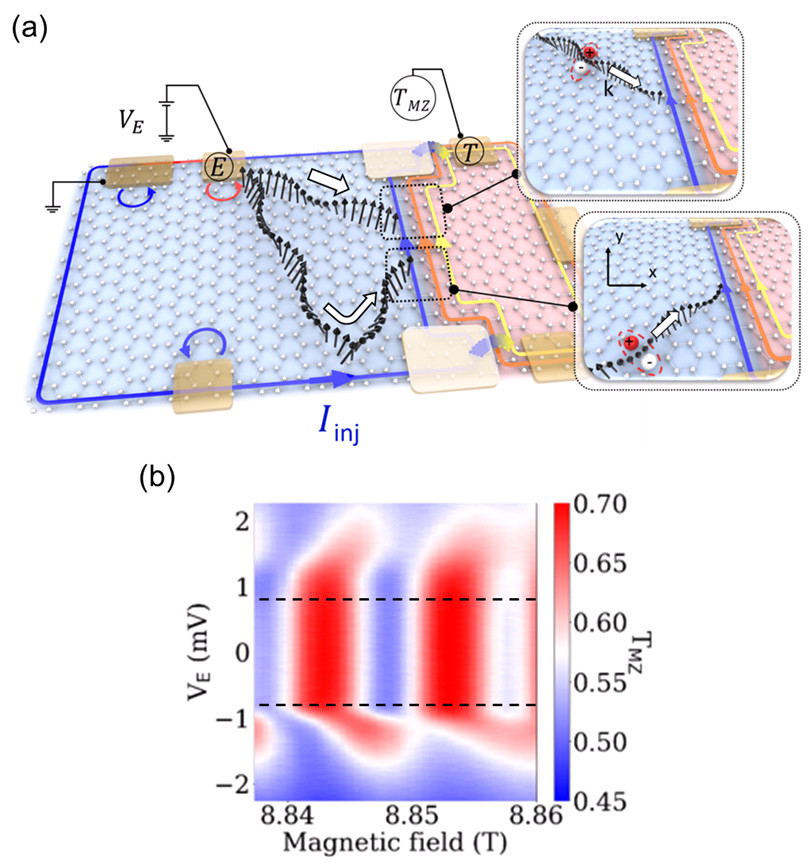
(a) Artist sketch of the experiment: a flake of graphene is divided into two regions with opposite p- and n- doping (blue and red areas), creating at their interface an electronic Mach-Zehnder between the edge channels of each region with same spin (blue and yellow arrows). An additional channel with opposite spin (red arrow) also propagates in the right region but does not play any role here. Magnons (wavy black arrows) are emitted from a biased contact Ⓔ towards the interferometer. Depending on the propagation direction relative to the interface (symbolized by the large white arrows), the magnons’ electric dipoles (red and white balls) can have different orientations when impinging on the interface, as depicted in the insets.
(b) Electronic transmission TMZ across the interferometer, measured versus bias voltage VE and magnetic field. Above the bias voltage threshold (black dashed lines), magnons are emitted and the interference patterns become tilted and significantly blurred, indicating phase shift and decoherence.
The researchers have confirmed this dual effect in their experiments (see Figure 1b), demonstrating the first observation of the excitonic nature of magnons in a quantum Hall ferromagnet. Furthermore, the analysis of their results unveils several elusive properties of the magnons; amongst them, the facts that the emission process is Poissonian (that is, magnons are indeed emitted randomly in time), and that increasing the drain-source potential increases the magnons’ emission rate rather than their energy.
The ability to couple magnetic excitations to quantum coherent conductors offers many exciting possibilities for experiments probing new phenomena at the intersection of mesoscopic quantum physics and spintronics.
Contact CEA: François Parmentier and Preden Roulleau, NanoElectronics Group (SPEC/GNE)
Publication:
Excitonic nature of magnons in a quantum Hall ferromagnet
A. Assouline, M. Jo, P. Brasseur, K. Watanabe, T. Taniguchi, T.Jolicoeur, D.C. Glattli, N. Kumada, P. Roche, F. D. Parmentier, & P. Roulleau, Nat. Phys. 17, 1369–1374 (2021) – arXiv:2102.02068.
Collaboration:
- Service de Physique de l’Etat Condensé – SPEC, UMR 3680 CEA-CNRS, Université Paris-Saclay
- Institut de Physique Théorique – IPhT, UMR 3681 CEA-CNRS, Université Paris-Saclay
- National Institute for Materials Science (NIMS), Japan
- NTT Basic Research Laboratories (NTT-BRL), Japan.