Since the early days of ultrafast science, the Laboratoire Interactions DYnamiques et Lasers (LIDYL) has been developing ever more powerful pulsed laser sources to explore dilute and condensed matter on ever finer time scales, reaching the attosecond range (1 as=10-18 s).
In the early 90s, the first technological revolution in this field was the advent of Titanium:Sapphire lasers, combined with Frequency Drift Amplification (CPA)*. This type of laser, enabling attosecond pulses to be generated by frequency conversion, has become widespread in laboratories studying ultrafast dynamics, and LIDYL’s ATTOLab platform has been built around these devices.
In recent years, the performance of lasers based on ytterbium (amplifier medium: KGd(WO4)2 crystal, doped with Yb3) has progressed significantly: coupled with temporal post-compression techniques, these laser sources are now competing with Ti:Sapphire lasers (Al2O3 sapphire crystal, doped with Ti3) and opening up a new era in attophysics. As part of its drive to remain at the cutting edge of performance, the ATTOLab platform is carrying out a major migration to these systems by 2024: four attosecond light lines driven by Ytterbium lasers will replace the existing Ti:Sapphire lines, with improved performance in terms of repetition rate (40 to 100 kHz) and reliability, combined with lower operating costs and energy efficiency of the entire laser chain, 6 times greater than that of Ti:Sapphire lasers.
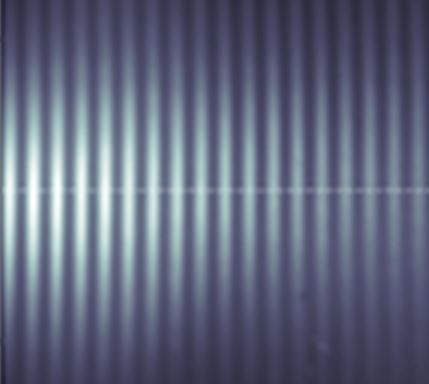
Attosecond physics and chemistry explore electronic dynamics in atoms (photoionization), molecules (chemical reactions) or condensed matter (solid state physics). Today, they are based on laser sources capable of delivering ultra-short light pulses in the attosecond range (1 as = 10-18 s), a time range characteristic of the dynamics of electronic structures.
The spectral width of a light pulse is inversely proportional to its duration: for a duration of 100 as, this width is greater than 18 eV, i.e. more than ten times the width of the visible spectrum! It is then made up of numerous coherent spectral components, which can be obtained by High Harmonic Generation (HHG). Harmonics are obtained by extremely non-linear conversion of laser pulses lasting tens of femtoseconds in the visible or infrared range, focused on a target of rare gas atoms. The resulting coherent spectrum is very broad, extending from the visible to the eXtreme UltraViolet (X-UV), with photon energies of several tens of eV, or even several hundred eV. This phenomenon was discovered at CEA in Anne L’Huillier’s group in the late 1980s. It is now used to generate almost all laboratory attosecond sources.
For GHOE’s highly non-linear effect to be optimal, initial femtosecond laser pulses with high illumination at the focal point, of the order of a few1014 W/cm2, are required. For this value, the laser electric field is of the order of the electric field that binds the electron to the nucleus in the atoms of the gas used for GHOE: He:1011 V/cm or Ar: 4×1010 V/cm. (Note that to maintain good generation efficiency, it remains advantageous to reach this illumination without reducing the focal spot size too much beyond ~20 to 100 µm).
Ti:Sapphire lasers have thus become the preferred choice, since they feature a gain curve with a very wide spectral range, enabling pulses of a few tens of fs to be generated. What’s more, they can be used to amplify pulse energy using the CPA technique, enabling the required illumination levels to be achieved after focusing.
Over the past ten years, an alternative technology to the Ti:Sapphire laser has emerged, based on a gain medium doped with Ytterbium (Yb3) , which is remarkably more efficient. Their “quantum defect”, i.e. the difference between pump and emission wavelengths, is much smaller, guaranteeing greater energy efficiency and less wastage in the form of material heating. Initially confined to the production of relatively low-energy pulses (~ 100 μJ/pulse), the performance of commercial devices is steadily increasing, with three of the systems to be fitted to the ATTOLab platform offering up to 2 mJ/pulse and 80 W average optical power. Their energy and shot-to-shot stability is also superior, by a factor of 2 to 3, increasing the sensitivity of experiments, particularly in absorption and transient reflectivity. Their repetition rate is much higher (40 and 100 kHz) and tunable. What’s more, their footprint is very compact, on the order of 0.2m2.
The final “hurdle” to be overcome in using these lasers has been the subject of intense research over the past ten years: the need to reduce pulse duration, which is far from ideal for GHOE (from a few hundred femtoseconds to picoseconds, much longer than that of Ti:Sapphire lasers, due to a narrower gain band). Thanks to an effort led by various laboratories, including the Laboratoire Charles Fabry (LCF) of the Institut D’Optique Graduate School (IOGS)
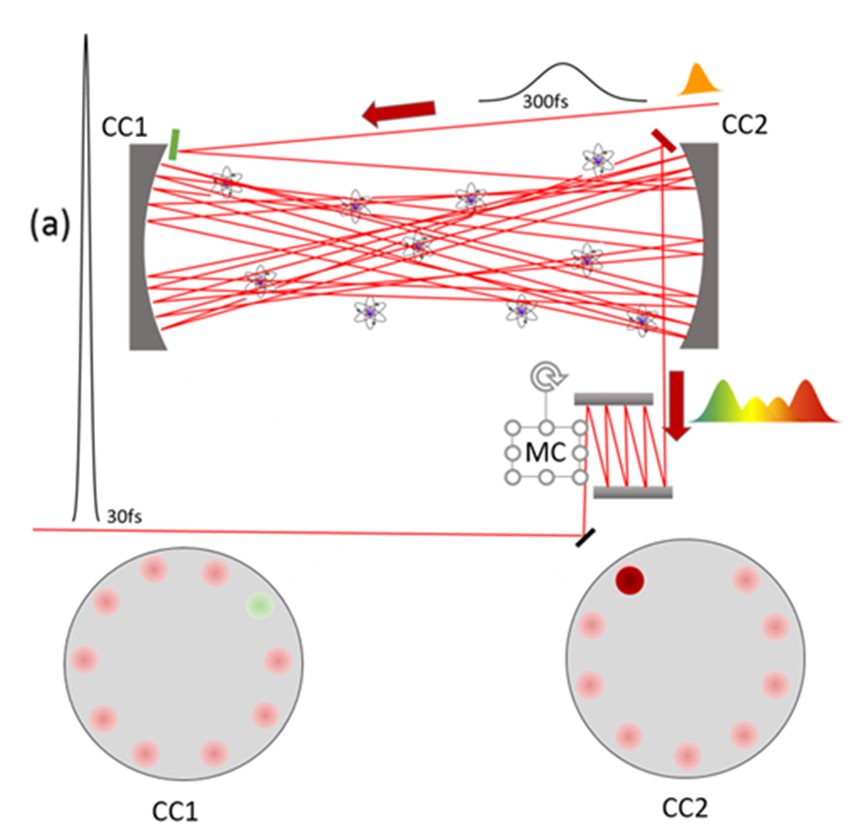
Ti: Sapphire |
Yb3-doped laser ATTOLab I (after post-compression) |
Yb3-doped laser ATTOLab II (after post-compression) |
|
Wavelength |
800 nm |
1030 nm |
1030 nm |
Average power | ~20 W |
80 W |
80 W |
Peak power per pulse |
80 GW |
26 GW |
66 GW |
Pulse duration | 25 fs | 30 fs | 30 fs |
Carrier/envelope phase stability (φ CEP) |
350 mrad |
Unstabilized |
250 mrad |
Repetition rate |
10 kHz |
100 kHz |
40 kHz |
Energy | 2 mJ |
0.8 mJ |
2 mJ |
Demonstration experiments carried out at LIDYL, as part of a technology watch in collaboration with the Charles Fabry Laboratory and the FastLite and Amplitude companies
The launch of the LUMA PEPR, of which LIDYL is a central experimental platform, and the award of two ERC Starting Grant grants to young LIDYL recruits, have made it possible to take this turn, which has been anticipated and planned for several years. Thus, by mid-2024, four Yb3 lasersand their associated post-compression cells, cumulating 260 W of useful optical power, with pulses in the 30 fs duration range, will be installed and optimized by the ATTO, DICO and SLIC groups working jointly on this project.
References
Marc Hanna, Florent Guichard, Nour Daher, Quentin Bournet, Xavier Délen, Patrick Georges, Lasers and Photonics Reviews, 15(12) (2021) 2100220.
Louis Daniault, Zhao Cheng, Jaismeen Kaur, Jean-François Hergott, Fabrice Réau, Olivier Tcherbakoff, Nour Daher, Xavier Délen, Marc Hanna, and Rodrigo Lopez-Martens, Optics Lett. 46(20) (2021) 5264.
Aura Inés Gonzalez, Gaëtan Jargot, Philippe Rigaud, Loïc Lavenu, Florent Guichard, Antoine Comby, Thierry Auguste, Olivier Sublemontier, Michel Bougeard, Yoann Zaouter, Patrick Georges, Marc Hanna, and Thierry Ruchon, JOSA B 35(4) (2018) A6-A14.
________________________________________
Contacts CEA-IRAMIS :
- Thierry Ruchon(LIDYL/ATTO)
- Jean-François Hergott(LIDYL/SLIC)
Collaborations :
Ti: Sapphire |
Yb3-doped laser ATTOLab I (after post-compression) |
Yb3-doped laser ATTOLab II (after post-compression) |
|
Wavelength |
800 nm |
1030 nm |
1030 nm |
Average power | ~20 W |
80 W |
80 W |
Peak power per pulse |
80 GW |
26 GW |
66 GW |
Pulse duration | 25 fs | 30 fs | 30 fs |
Carrier/envelope phase stability (φ CEP) |
350 mrad |
Unstabilized |
250 mrad |
Repetition rate |
10 kHz |
100 kHz |
40 kHz |
Energy | 2 mJ |
0.8 mJ |
2 mJ |
Demonstration experiments carried out at LIDYL, as part of a technology watch in collaboration with the Charles Fabry Laboratory and the FastLite and Amplitude companies
The launch of the LUMA PEPR, of which LIDYL is a central experimental platform, and the award of two ERC Starting Grant grants to young LIDYL recruits, have made it possible to take this turn, which has been anticipated and planned for several years. Thus, by mid-2024, four Yb3 lasersand their associated post-compression cells, cumulating 260 W of useful optical power, with pulses in the 30 fs duration range, will be installed and optimized by the ATTO, DICO and SLIC groups working jointly on this project.
References
Marc Hanna, Florent Guichard, Nour Daher, Quentin Bournet, Xavier Délen, Patrick Georges, Lasers and Photonics Reviews, 15(12) (2021) 2100220.
Louis Daniault, Zhao Cheng, Jaismeen Kaur, Jean-François Hergott, Fabrice Réau, Olivier Tcherbakoff, Nour Daher, Xavier Délen, Marc Hanna, and Rodrigo Lopez-Martens, Optics Lett. 46(20) (2021) 5264.
Aura Inés Gonzalez, Gaëtan Jargot, Philippe Rigaud, Loïc Lavenu, Florent Guichard, Antoine Comby, Thierry Auguste, Olivier Sublemontier, Michel Bougeard, Yoann Zaouter, Patrick Georges, Marc Hanna, and Thierry Ruchon, JOSA B 35(4) (2018) A6-A14.
________________________________________
Contacts CEA-IRAMIS :
- Thierry Ruchon(LIDYL/ATTO)
- Jean-François Hergott(LIDYL/SLIC)
Collaborations :