A collaboration of physicists has developed a new type of superconducting quantum bit at low frequencies (MHz instead of GHz), whose sensitivity to electrical charges enables the quantum state of a mechanical micro-resonator to be controlled and measured.
The device provides a component that could enable advances in a new physics combining gravitation and quantum mechanics.
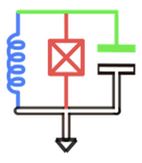
Detecting the quantum vibrations of a mechanical resonator, or even preparing such a massive object in a quantum superposition state where it simultaneously occupies two distinct positions, requires being able to measure and control the system with a device that is itself quantum, such as a superconducting qubit. Qubits, developed for quantum information, would be well suited, if they did not operate at frequencies well above (a few GHz) those of mechanical micro-resonators of the MEMs type developed in the laboratory, whose resonance frequency is of the order of MHz.
By improving a device called fluxonium, a collaboration of physicists has succeeded in manufacturing a superconducting qubit operating at the same frequency as these mechanical micro-resonators. It has also been shown that this fluxonium is also a highly sensitive electric charge sensor. The ability to reduce the frequency of superconducting qubits by a factor of 1000, and make use of their extreme sensitivity to electrical charge fluctuations, provides a decisive element for future experiments on massive quantum systems. Such a sensor could enable advances in new physics combining gravitation and quantum mechanics .
Superconducting qubits are a system of choice as an information carrier in the quantum industry. These are systems whose basic component is a Josephson junction, made with two layers of superconducting material separated by an insulating layer of metal oxide, through which electrons can tunnel. These systems transit between two discrete states when excited at a specific frequency. In the superconducting qubit transmons used in industrial quantum computers (Google, IBM, Amazon, etc.), these transitions occur in the microwave range (a few GHz), close to the frequency of cell phone signals. However, mechanical resonators capable of storing quantum information for long periods of time have a frequency a thousand times lower (MHz range). In this work, researchers have succeeded in extending the operational limits of a qubit below 2 MHz.
The resulting system is called heavy fluxonium. It consists of a Josephson junction, a capacitor (metal electrodes separated by an insulator), and a superinductance. This last component is the key to the device, as it eliminates electrical noise at these low frequencies, which is omnipresent in the environment and highly problematic in quantum systems of this type. It consists of a chain of 360 Josephson junctions, producing an inductance of 2 µH over a surface area of just 0.002 mm². The two discrete states of this qubit correspond to “permanent currents”, flowing through the superconducting loop in one direction or the other. The energy difference between these two states is adjustable and depends on the value of the external magnetic field applied to the loop.
Moreover, at a specific value of the magnetic field, the qubit states consist of a “chat-de-Schrödinger” superposition of the two permanent current states in opposite directions. At this operating point, this work demonstrates that the qubit is highly sensitive to an applied electric field.
So, in addition to its very low frequency, the fluxonium produced in this work is an electric charge sensor whose sensitivity equals that of the best existing systems. The sensitivity achieved is 33 µe/Hz1/2, which means that in one second it can detect an electric charge variation of 33 millionths of an electron. This crucial advance could then be exploited to detect the quantum vibrations of a mechanical micro-resonator (a kind of micro-membrane drum) carrying electrical charges and oscillating in the vicinity of the fluxonium, the two systems being capacitively coupled.
In the medium term, by combining the extreme sensitivity of this circuit to electric charge modulation, and its low operating frequency, it is conceivable to prepare a micro-drum, in a quantum superposition for which the drum membrane simultaneously occupies two distinct positions. As this object has mass, it generates a gravitational field, the true origin of which is still unknown. This work paves the way for experiments that could elucidate this age-old debate surrounding one of the major unresolved questions in modern quantum physics.
Reference:
High-sensitivity AC-charge detection with a MHz-frequency fluxonium qubit.
Baldo-Luis Najera-Santos, Rémi Rousseau, Kyrylo Gerashchenko, Himanshu Patange, Angela Riva, Marius Villiers, Tristan Briant, Pierre-François Cohadon, Antoine Heidmann, José Palomo, Michaël Rosticher, Hélène le Sueur, Alain Sarlette, William Clarke Smith, Zaki Leghtas, Emmanuel Flurin, Thibaut Jacqmin & Samuel Deléglise, Phys. Rev. X 14 (2024) 011007.
- See also the “highlight” from the editor of Physics Magazine:“Superconducting qubit breaks low-frequency record“.
- arXiv preprint arXiv:2307.14329
- See CNRS news:“Un qubit superconducteur comme capteur ultrasensible dans le domaine radiofréquence“.
CEA-SPEC contact: Emmanuel Flurin and Hélène Le Sueur, IRAMIS/SPEC/GQ
Collaboration :
- Sorbonne University, Laboratoire Kastler Brossel, CNRS, ENS, Université PSL, and Collège de France,
- Université Paris-Saclay, Équipe Quantronique du SPEC (CEA), UMR CEA-CNRS
- Quantic team (INRIA),
- LPENS (ENS, Sorbonne University, Université PSL, CNRS)
- Alice&Bob
This work was carried out with funding from the ANR Mecaflux project (2021-2025), coordinated by Samuel Deléglise (Laboratoire Kastler Brossel), a partnership between Inria Paris, LPENS Laboratoire de physique de l’ENS, LKB – Laboratoire Kastler Brossel and SPEC Service de physique de l’état condensé.