Presentation
Complementary to micro X-ray diffraction, the Raman microspectrometry funded by the ESPADON project is an analytical device of critical importance for characterising the crystalline structure of materials. As such it is well suited to determining the distribution of phases formed in the corrosion layers of iron or steels over decades or centuries in varying environments. Iron oxides, oxy-hydroxides and carbonates are the main phases encountered in these systems at LAPA, studied in transverse sections.
Mapping acquisitions are well suited to answer to these questions (Figure). The exploitation of these data is conducted following different methods with the help of the Wire software developed by Renishaw. The first one is based on the Region of Interest (Signal to baseline methods). But the dataset can also be exploited by supervised or unsupervised multi-variate analyses.
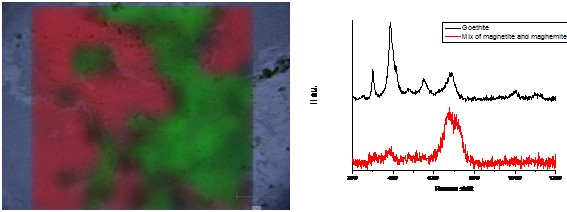
Controlled laboratory experiments allow us to better understand corrosion mechanisms. For example, deuterium solutions can be used to re-corrode materials, in order to identify the location of precipitated corrosion phases on archaeological artefacts. Raman microspectrometric analyses of the deuterated carbonated phases at the interface between metal and its corrosion products allows us to comprehend long-term ferrous corrosion mechanisms in an anoxic environment. The electrochemical behaviour of phases present inside these corrosion layers has been explored with an electrochemical cell set up under the Raman microspectrometer. For example, maghemite put under a cathodic potential is reduced to magnetite and reoxidised to maghemite when aerated conditions are reconducted.
LAPA’s Raman spectrometer is a Renishaw Invia reflex. It is equipped with a frequency-doubled Nd: YAG emitting at 532 nm and a solid-state laser emitting at 473 nm. The laser beam is focused on the sample through an optical microscope and a x50, long working distance x50 and a x100 LEICA objectives. The laser spot size is less than 2 µm. A 2400 l/mm grating induces a spectral resolution of about 1 cm-1. The detector is a charge-coupled devicemultichannel matrix detector operating at −70° C (by air cooling). The laser beam power on the surface of the sample can be modulated using optical density filters. Thanks to a motorized stage, mapping acquisitions can be collected. The hyperspectral images obtained provide fruitful information on the location of various crystalline phases constituting the observed systems.
Neff, D., L. Bellot-Gurlet, et al. (2006). “Raman imaging of ancient rust scales on archaeological iron artefacts for long-term atmospheric corrosion mechanisms study.” Journal of Raman Spectroscopy 37(10): 1228-1237.
Neff, D., S. Reguer, et al. (2004). “Structural characterization of corrosion products on archaeological iron. An integrated analytical approach to establish corrosion forms.” Journal of Raman Spectroscopy 35: 739-745.
Reguer, S., D. Neff, et al. (2007). “Deterioration of iron archaeological artefacts: micro-Raman investigation on Cl-containing corrosion products.” Journal of Raman Spectroscopy 38(4): 389-397.
Saheb, M., D. Neff, et al. (2010). “Raman study of a deuterated iron hydroxycarbonate to assess long-term corrosion mechanisms in anoxic soils.” Journal of Raman Spectroscopy: n/a-n/a.