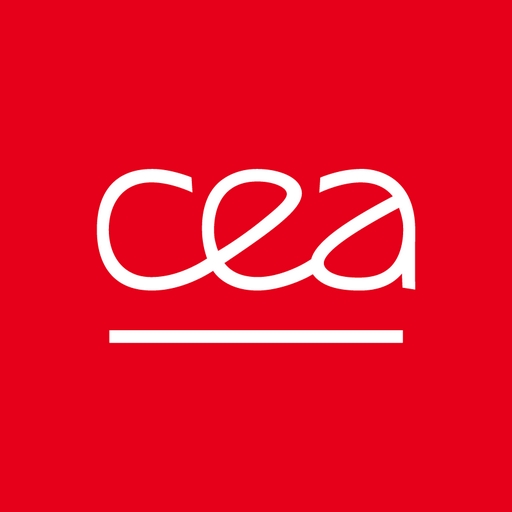
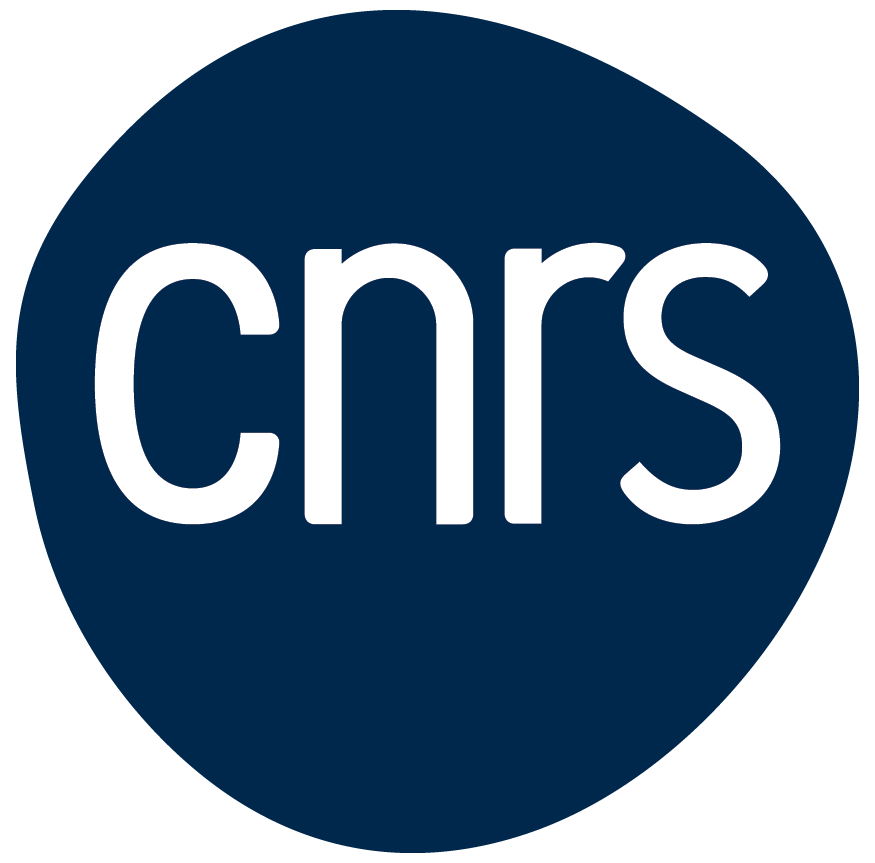
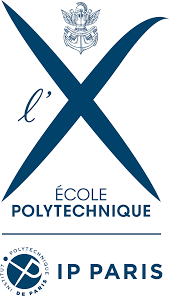
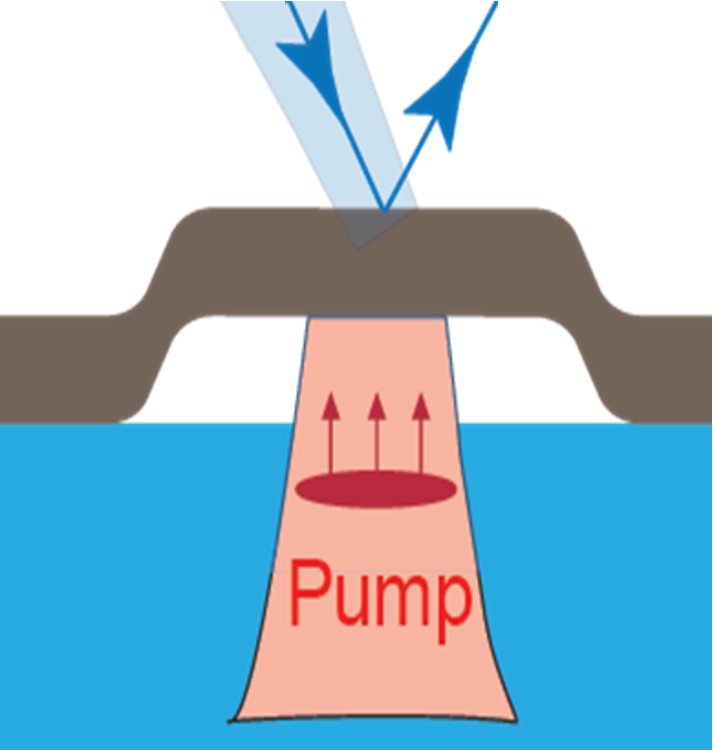
Femtosecond laser pulses are commonly used to study or design new structures for a wide range of applications in nanotechnology, chemistry, solid-state physics, life sciences, and today in industrial processes (e.g. micromachining). At low energies, these ultrashort pulses can excite ultrafast dynamics in the samples under study, or, at higher intensities, induce stable modifications creating new nanostructures with unique physical properties.
Pavel Varlamov’s PhD thesis [1] and an associated publication in Nanomaterial [2] describe a process for building micrometer-sized cavities, obtained by delamination induced by a single high-energy femtosecond laser pulse. The phenomenon is observed on metallic thin films and ferromagnetic multilayers [3].
Low-energy laser beams, both pulsed and continuous, can be used to characterize these nanostructures almost in situ, using a range of non-destructive nano-optical techniques: interferometric microscopy, ultrafast photoacoustics and magneto-plasmonics [3].
Nanostructures can be produced from thin-film or multilayer samples by delamination, i.e. local separation of the layers from the substrate. The principle of femtosecond laser delamination (FLS) is quite simple: single laser pulses are focused onto the thin film or multilayer through an optically transparent substrate. The film thickness and laser focusing conditions (laser fluence) are chosen so that only the central part of the excited film separates from the substrate, forming closed cavities. Depending on laser focusing and fluence (incident laser energy per unit surface area, measured in J/cm2), their size ranges from a few hundred nanometers to several microns. The cavity structure thus formed can be further characterized by optical interference microscopy during the laser nanofabrication process.
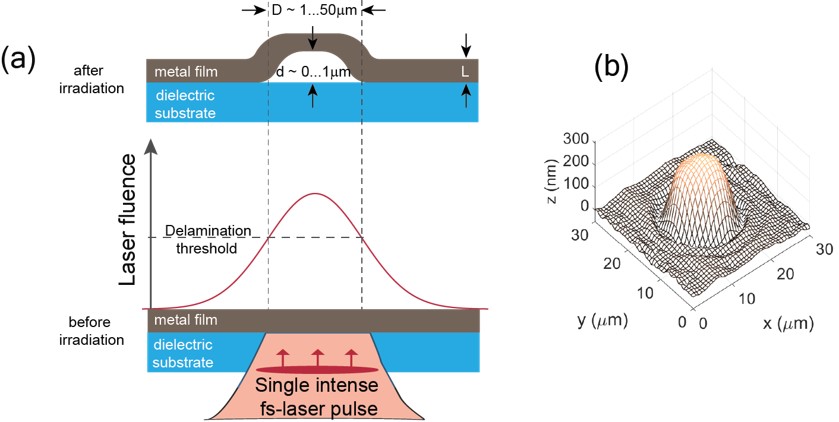
Fig. 1 (a) A single high-energy femtosecond laser pulse with peak fluence > 0.1 J/cm2 focused on a thin metal film (or multi-layer structure) produces a closed “femtosecond laser delamination -DLF” cavity. (b) The resulting cavity shapes can be observed by in situ optical interferometric microscopy, integrated with the nanofabrication device.
The physical properties of the cavities obtained by FLD are noteworthy: the delaminated part of the membrane forms a high-quality “acoustic” cavity. Time-resolved photoacoustic microscopy using low-energy laser pulses (< 1/100th that of the high-energy pulses creating the structure) allows to record pulses of coherent acoustic phonons propagating in the membrane. Analysis of the dynamics of the modes thus recorded provides access to the properties of these ultra-high-frequency phonon modes, higher than 100~GHz [1,2].
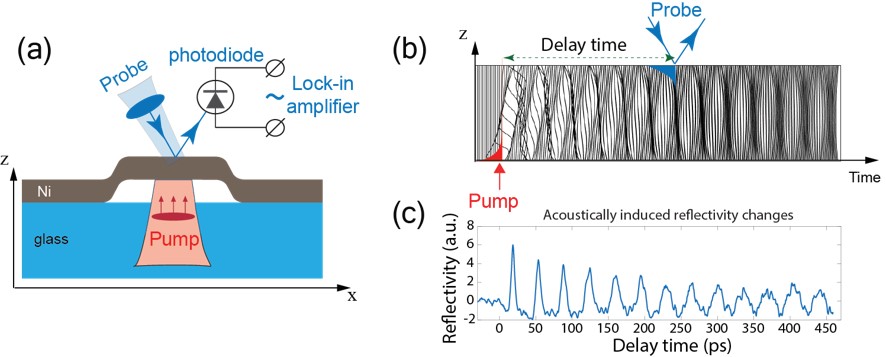
Fig. 2: Using low-energy pulses, time-resolved pump-probe microscopy can be used to measure the ultrafast dynamics in FLD cavities, obtained by laser delamination of a thin layer of nickel on a glass substrate. (b) Elastic vibrations are confined within each FLD cavity, as shown in (c) by multiple peaks in the time-resolved reflectivity signal.
Another system based on cobalt-gold bilayers on sapphire shows that the cavities obtained by FLD possess interesting plasmonic properties (electronic collective oscillations). The separation obtained between the cobalt-gold bilayer and the substrate is of the order of an optical wavelength, i.e. ~ 500 nm. This configuration is a realization of the “Otto configuration”, in which the light is focused onto the thin metal layer through a dielectric prism. Optimum excitation of surface plasmon polaritons (SPPs)* in the visible spectral range is achieved by separating the metal layer from the prism by the distance of the order of a micron. The delamination effect separating the metal layer from its support thus provides a simple, optimal configuration for plasmonic excitation.
A magneto-plasmonics experiment shows that it is also possible to control the SPP excitation by applying weak external magnetic fields of the order of a few milliTesla [1,3]. The reflectivity is thus a function of the angle and of the state of magnetization in the direction perpendicular to the plane of incidence of the light (transverse magneto-optic Kerr effect or T-MOKE). The Figure 3 illustrates the result obtained.
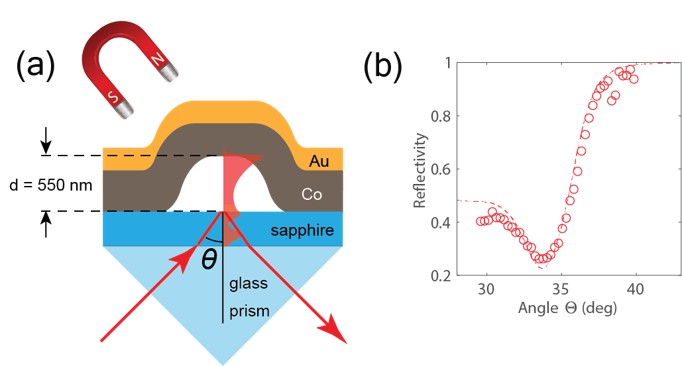
Fig 3: a) A high-intensity femtosecond laser pulse (peak fluence ~0.5 J/cm2) forms a FLD cavity by separating the Au/Co bilayer from the sapphire substrate, the whole assembly being mounted on a rotating prism. b) The reflectivity minimum as a function of the angle of incidence gives the excitation condition for the resonance associated with the excitation of Surface Plasmons-Polaritons (SPP), also a function of the applied magnetic field.
The obtained experimental results, supported by their numerical modeling [3], reveal the potential of the DLF cavity process, using all-optical techniques, to build and characterize active magneto-plasmonic devices operating at ultrafast timescales. Applied to magnetic thin films, the characterization method combines the advantages of femtosecond laser excitation, ultra-high-frequency acoustic vibration analysis of nanostructures and magnetization sensitivity.
* A surface plasmon-polariton is a particular mode of collective electronic oscillation (plasmon) that propagates parallel to the surface.
References:
[1] Pavel Varlamov PhD thesis: “Femto-second-laser produced nanostructures for advanced magnetic, acoustic and plasmonic applications”, P. , PhD Thesis, École Polytechnique, IP Paris (2025)
[2] Femtosecond laser ablation and delamination of functional magnetic multilayers at the nanoscale,
Pavel Varlamov, Jan Marx, Yoav Urbina Elgueta, Andreas Ostendorf, Ji-Wan Kim, Paolo Vavassori, and Vasily Temnov, Nanomaterials 14 (2024) 1488.
[3] “Grazing incidence nanogap resonance in the prism-gap-ferromagnet magneto-plasmonic Otto configuration” P. Varlamov, A. Barros, A. Swaminathan, A. Lomonosov, M. Raynaud and V.V. Temnov, Opt. Lett. 50, 109 (2025).
Contact CEA-IRAMIS: Vasily Temnov – LSI/ Équipe PC-NANO
Collaborations:
- Laboratoire des Solides Irradiés – LSI UMR CEA-CNRS-École Polytechnique, Institut Polytechnique de Paris, 91128 Palaiseau, France
- A. Ostendorf, Applied Laser Technologies, RUB Bochum, Germany
- CIC nanoGUNE—BRTA, Donostia—San Sebastian, Spain
- Department of Polymers and Advanced Materials: Physics, Chemistry and Technology, University of the Basque Country 20018 Donostia-San Sebastián, Spain
- Kunsan National University, Kunsan-si, Jeonbuk, Korea.
- IKERBASQUE, Basque Foundation for Science, 48009 Bilbao, Spain