The operation of conventional electronic devices use accumulation (electric potential) and electric charge currents carried by electrons. Spintronics, on the other hand, leverages the other property of electrons—their spin or intrinsic angular momentum.
Spintronics paves the way for faster and more efficient electronic devices than those available today. However, for this technology to advance, an efficient and rapid method of generating spin polarizations and spin currents is needed—meaning organized flows of electrons with aligned spins that can be used to encode information in magnetic materials.
Using lasers to create spin currents is an option explored by many research groups, but until now, the degree of control available has been limited. In current approaches, the laser provides energy to the material, which is then redistributed through spin currents, primarily governed by electronic diffusion.
An international collaboration involving the DICO team from LIDYL has observed that a laser pulse can directly induce spin currents, and this occurs very quickly—in just a few femtoseconds. This finding suggests new methods for more flexible and precise control of spin currents in spintronics.
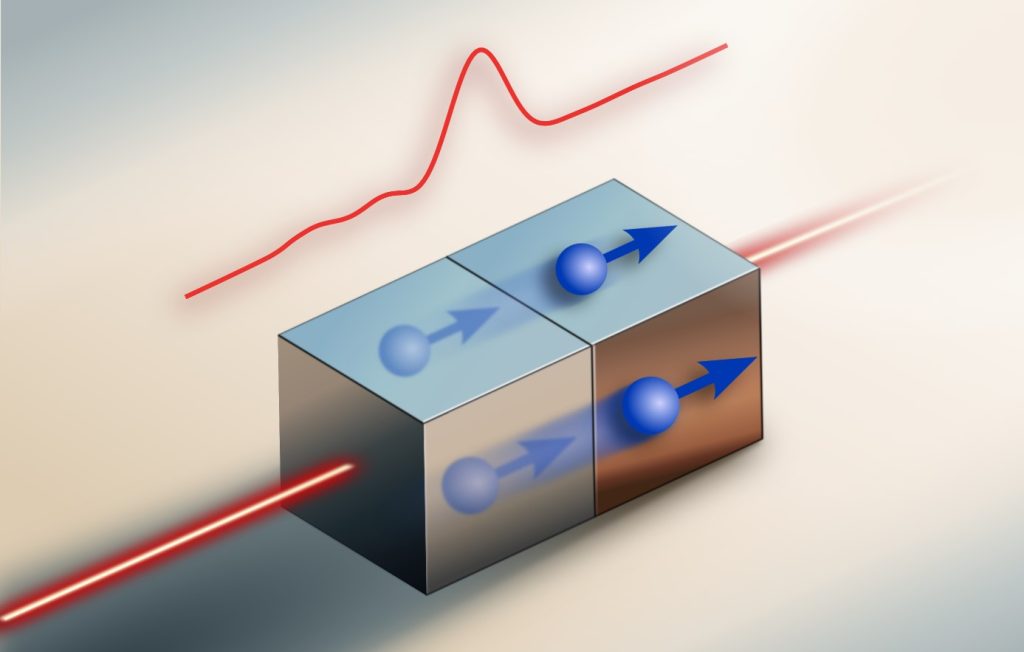
The magnetization of materials can be altered by a femtosecond laser pulse (~ 10-15 s), which forms the basis of a research field known as femtomagnetism. In a ferromagnetic solid, the spins of electrons are ordered: they align parallel to the direction of an external magnetic field. When subjected to a laser pulse, electrons gain energy and begin to spatially diffuse and transition between the material’s energy levels. During these processes, some of their spin orientation is lost, leading to a reorganization of magnetization within the material, which generates a spin current (without necessarily causing a net charge current). This process is extensively studied because it offers possibilities for controlling magnetic memories and interfacing information storage and transport with light.
Current methods rely on incoherent phenomena, such as electron diffusion. The time required for these phenomena is intrinsic to each material and ranges from about 100 femtoseconds to 10 picoseconds. Since this time is almost independent of the shape or duration of the excitation laser pulse, control possibilities remain fairly limited, and operational frequencies are constrained by the material itself.
In this experiment, we conduct one of the first measurements of spin dynamics on the attosecond scale. The measurement is performed on a sample consisting of 20 alternating layers of cobalt and platinum, each about 1 nm thick. This thin-layer alternating pattern of two different metals presents multiple interfaces where symmetry is broken, giving rise to spintronic effects.
The structure is first excited by a very short laser pulse (less than 4 femtoseconds), which triggers a reorganization of spins. Immediately afterward, the sample is probed by an even shorter pulse (attosecond duration) that is circularly polarized. This allows to measure a quantity called magnetic circular dichroism, which provides information about the spin state in each of the two metals. The significant advance of this study is the ability to measure this quantity for cobalt and platinum simultaneously, with a temporal resolution in the attosecond range (~ 10 -18 s).
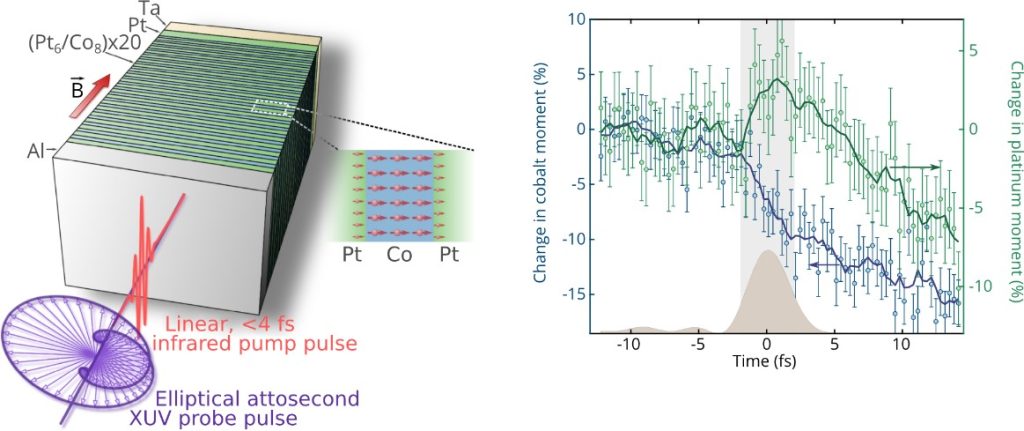
Attosecond pump-probe experiment on a spintronic structure. The pump pulse (excitation) is linearly polarized, while the probe pulse, delayed by a variable time of a few femtoseconds, is circularly polarized to obtain a circular dichroism signal. The platinum and cobalt layers are represented in green and blue, respectively.
Spin dynamics in cobalt (blue) and platinum (green). The laser pulse is shown in brown. We see that during the laser pulse, the spins behave oppositely in the two metals, indicating a spin transfer at the interface.
The data show that the laser induces a sudden change in the magnetic order within the sample. During the laser pulse, the magnetization of the cobalt layers decreases very rapidly—about 10% in 2 femtoseconds, which is much faster than previously measured. Even more surprising, the magnetization of the platinum layers simultaneously increases by several percent. This behavior is extremely well reproduced by simulations performed using time-dependent density functional theory (TD-DFT). Together, the experiment and theory suggest that the laser triggers a spin transfer from cobalt to platinum. Remarkably, this spin transfer occurs only during the laser pulse, which lasts only a few femtoseconds. Thus, the observed effect is fundamentally different from what has been known so far: the shape and duration of the spin current are directly dictated by the laser pulse, rather than by the material and electronic diffusion.
Beyond observing an ultra-short spin transfer, these results pave the way for new schemes to control spintronic functionalities. Indeed, it is relatively easy to shape the form, polarization, or duration of laser pulses. By using the mechanism demonstrated here, it becomes possible to control spin currents, which are privileged information vectors, more precisely and rapidly.
Référence :
[1] “Spin Dynamics across Metallic Layers on the Few-Femtosecond Timescale “
R. Géneaux, H.-T. Chang, A. Guggenmos, R. Delaunay, F. Légaré, K. Légaré, J. Lüning, T. Parpiiev, I. J. P. Molesky, B. de Roulet, M. Zuerch, S. Sharma, M. Schultze et S. R. Leone, Phys. Rev. Lett 133, 106902 (2024)
HAL Science ouverte : .https://cea.hal.science/cea-04693950.
Contact CEA-IRAMIS : Romain Géneaux (DRF/IRAMIS/LIDYL/DICO)
Collaboration :
- M. Schultze : Institute of Experimental Physics, Graz University of Technology, Petersgasse 16, 8010 Graz, Austria
- H.-T. Chang, A. Guggenmos, T. Parpiiev, I. Molesky, B. de Roulet, M. Zuerch, S. Leone Department of Chemistry, University of California, Berkeley, California 94720, USA
- R. Delaunay : Sorbonne Université, CNRS, Laboratoire de Chimie Physique-Matière et Rayonnement, LCPMR, 75005 Paris, France
- F. Légaré, K. Légaré : Institut National de la Recherche Scientifique, Centre Énergie Matériaux et Télécommunications, Varennes, Québec, Canada
- J. Lüning : Helmholtz-Zentrum Berlin für Materialien und Energie, Albert-Einstein-Strasse 15, 12489 Berlin, Germany
- S. Sharma : Max-Born-Institute for Non-linear Optics and Short Pulse Spectroscopy, Max-Born Strasse 2A, 12489 Berlin, Germany