Lightweight, inexpensive plastics are often preferred as container materials for food, household and pharmaceutical products. Active research is still required to ensure that the chosen plastic is inert with respect to the contents and will not deteriorate their qualities. This is all the more important for pharmaceutical products, which contain proteins that degrade more easily.
A study carried out by two teams from NIMBE and I2BC at CEA, in association with INRAE and IMMM, shows that solubilized proteins can be destabilized by contact with the walls of the bottle, leading to the formation of protein aggregates at the interface with the air, and then in solution. Plastic is the most unfavorable material, compared with glass or Teflon.
The study goes on to examine the role played by the presence of microplastic in biological media.
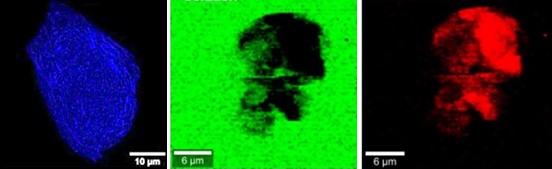
The destabilization and aggregation of proteins in biotherapeutic products (particularly new monoclonal antibodies for therapeutic or diagnostic purposes) can reduce their activity and efficacy. It can also promote immune reactions responsible for serious adverse effects. The impact of bottling and transport methods on protein destabilization is not fully understood, and active research on this subject is ongoing.
The I2BC and NIMBE teams at CEA, in association with IMMM and INRAE, have therefore combined their skills to study in depth
Observations combine protein loss monitoring (with UV-visible spectroscopy and Raman micro-spectroscopy), coupled with dynamic light scattering measurements (using laser “speckle” analysis) and proteomics (study of the proteome, the set of proteins contained in the system, by mass spectrometry). The study shows that flask materials, in particular plastics, play an initiating role in the destabilization of the proteins they contain, similar to what was previously observed by the same teams on silica
This set of measurements has also enabled us to propose a mechanistic model for protein destabilization (see figure 1): proteins are destabilized on contact with the flask surface, and then display a hydrophobic function. Under the effect of agitation, they then migrate to the air-water interface, where they compact to reduce the interface length with the liquid, in the form of micron-sized protein aggregates (see figure 2). Finally, still due to agitation, surface shear forces release these aggregates back into solution. A portion of the surface then becomes free for the aggregation of new, destabilized proteins. Ultimately, the solution becomes heavily contaminated with aggregates of degraded proteins that have lost their functionality.
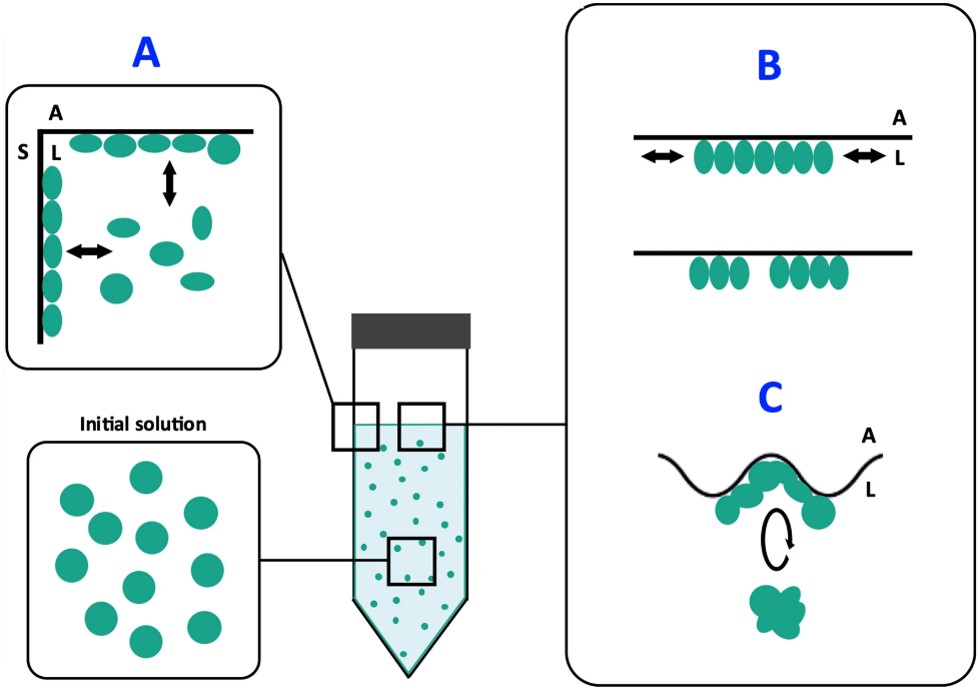
(A) Proteins in solution are destabilized by contact with the bottle wall.
(B) The proteins, now displaying a hydrophobic character, migrate and adsorb at the air-liquid interface, where agitation induces compression and decompression forces favoring their aggregation.
(C) Agitation-induced deformation of the interface induces shear forces that can rupture the protein films formed in step B, facilitating the transfer of aggregates into solution, with a possible return to step A.
The mechanism is strong enough to lead to the degradation of otherwise very robust proteins such as hemoglobin. However, it is the larger protein assemblies (several tens of nanometers) that are the most sensitive, probably due to capillary effects and surface pressure at the air-water interface.
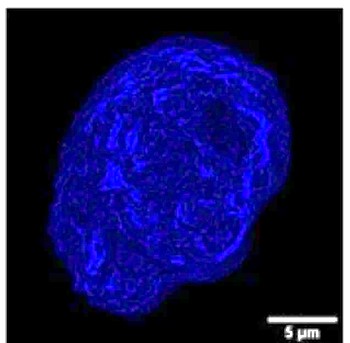
The study shows that polypropylene is the most unfavorable material for protein degradation. Teflon is less reactive, but more expensive. It should be noted, however, that even if the vial induces little degradation, the small quantity of protein degraded may be enough to trigger an undesirable immune reaction. Particular attention must therefore be paid to the packaging of protein products, especially for new therapies based on monoclonal antibodies.
These observations, and the proposed mechanistic model, also provide clues as to how to minimize these effects: by reducing or even eliminating one of the stress components – container material, air (even partially) or agitation – proteins will be better preserved. The teams are continuing their research by studying the impact of the presence of microplastics, which can be dispersed in a biological environment, on biomolecules.
References
M. Schvartz, F. Saudrais, S. Devineau, J-C Aude, S. Chédin, C. Henry, A. Millán-Oropeza, T. Perrault, L. Pieri, S. Pin, Y. Boulard, G. Brotons & J-P Renault. Scientific Reports 13 (2023) 1227.
D. Sanchez-Guzman, G. Giraudon–Colas, L. Marichal, Y. Boulard, F. Wien, J. Degrouard, A. Baeza-Squiban, S. Pin, J-P Renault, and S. Devineau, ACS Nano 14(7) (2020) 9073-9088.
See also:
-
The crown of proteins adsorbed on silica nanoparticles reveals its structure(on the Institut Joliot website).
- Theimportance of protein size in protein-nanoparticle interactions(on the Institut Joliot website)
Contacts
- CEA-IRAMIS: Jean-Philippe Renault (NIMBE/LIONS)
- CEA-JOLIOT: Yves Boulard(I2BC-S/SB2SM/LBSR)
Collaboration :
- M. Schvartz, F. Saudrais, S. Chédin, L. Pieri, S. Pin, J-P Renault, Université Paris-Saclay, UMR NIMBE CEA-CNRS, France
- F. Saudrais , J-C Aude, S. Chédin, L. Pieri, Y. Boulard, Université Paris-Saclay, UMR I2BC CEA-CNRS.
- M. Schvartz, T. Perrault, G. Brotons, Institut des Molécules et Matériaux du Mans (IMMM), UMR 6283 CNRS, Le Mans Université.
- C. Henry, A. Millán-Oropeza, Université Paris-Saclay, INRAE, AgroParisTech, Micalis Institute, Plateforme d’Analyse Protéomique de Paris Sud Ouest (PAPPSO).
- S. Devineau, Functional and Adaptive Biology Unit (BFA – UMR 8251 CNRS – Université Paris Cité).