In the space of just 20 years, rechargeable lithium-ion batteries have made their mark on the portable electronics market, thanks in particular to their improved performance thanks to the use of graphite anodes. The mechanism of their electrochemical activity is based on the intercalation of lithium between graphene planes, to which it transfers its two electrons. The maximum insertion capacity corresponds to the formation of the LiC6 compound, which is equivalent to a theoretical capacity of 372 mAh.g-1, still considered insufficient for application in electric vehicles. The search for new anode materials therefore remains very active. The conjugate insertion of Li with another electropositive metal (Ca, Eu, etc.) within graphite enables this stoichiometry to be exceeded (e.g. Li3.0-3.2Ca2.1-2.3C6).
The present study demonstrates the value of the concentration measurement methods offered by the NIMBE nuclear microprobe for studying the development of such ternary-phase electrodes.
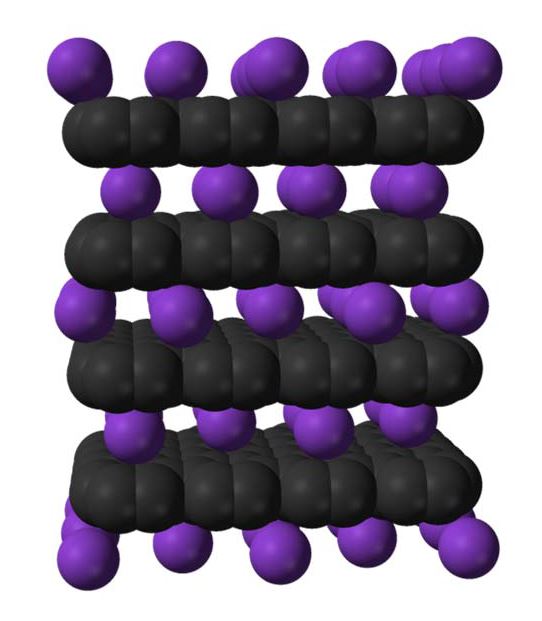
For lithium batteries, graphite anodes, in which lithium is inserted, are among the electrodes with low intercalation potential, helping to avoid the formation of lithium dendrites, a source of internal short-circuits. Such anodes also offer a good performance compromise between efficient lithium transport, good mechanical stability during charge/discharge cycles, high electrical conductivity and low cost. However, their capacity remains limited by the stoichiometry of the LiC6 compound, and improving the performance of Li-ion batteries today requires a search for new Graphite Intercalation Compounds (GICs), which are far from having all been identified.
A team from Nancy’s Jean Lamour University, led by Claire Hérold, is currently exploring the possibilities of obtaining new co-inserted ternary CIGs, where graphite is associated with an alkali metal and a strongly electropositive third element, or binaries, where lithium is this time used as the third element’s intercalation vector [1].
Achieving the joint intercalation of two elements is difficult. It can be achieved by immersing a block of pyrographite in a lithium-based molten alloy also containing alkaline earths (calcium) or rare earths (europium)
As lithium contributes very little to diffraction, determining the structural arrangement of the ternary compounds formed requires complete and accurate determination of their composition. This is made difficult by the simultaneous presence of the two light elements (lithium and carbon), and the heterogeneities associated with the synthesis method.
The stoichiometry of the α-phase (Li0.2Eu2C6.) was determined for the first time, using the nuclear microprobe of the Laboratoire d’Etude des Eléments Légers (LEEL) of the NIMBE (UMR 3685 CEA-CNRS). Nuclear microanalysis is one of the few local methods that can measure all these elements simultaneously and absolutely. As shown in Figure 1, the particle spectrum produced by a proton microbeam is the result of several interactions:
- elastic scattering, enabling measurement of carbon and europium concentrations (RBS method, Rutherford Backscattering Spectroscopy),
- nuclear reactions, to measure lithium concentration (NRA, Nuclear Reaction Analysis).
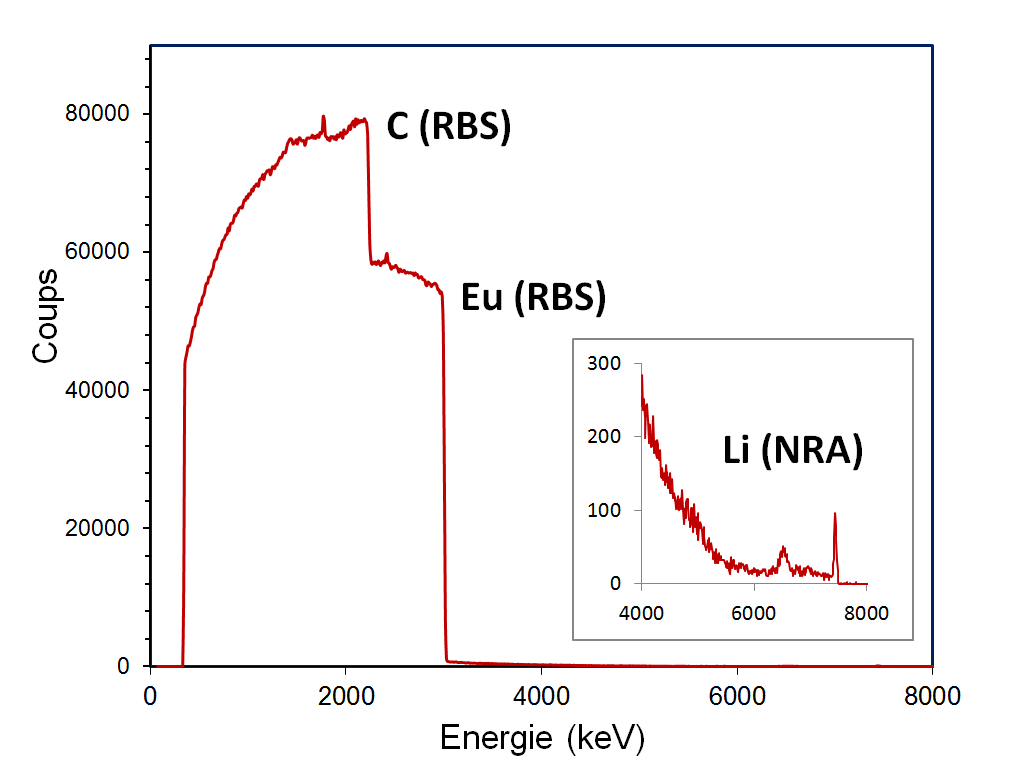
The stacking sequence of the α-phase was thus determined from these compositional measurements and X-ray diffraction spectra, supplemented by modeling of the electron density distribution perpendicular to the planes. The result is a structure in the form of a 5-layer sheet sequence, inserted between two graphene planes, comprising three europium planes flanked by two lithium planes.
However, this is not the only phase. It is interesting to note that immersion of pyrographite in a lithium-europium mixture does not systematically lead to the formation of this ternary phase, but may simply result in that of the EuC6 binary. However, the reaction begins with the progressive intercalation of lithium. An X-ray diffraction study of the kinetics of EuC6 formationfrom a 72% lithium Li/Eu mixture shows the initial formation of increasingly lithium-rich LiC compounds up to the LiC6 phase. After just a few hours, the LiC6 phase is replaced by EuC6 without passing through an intermediate ternary phase. The initial intercalation of lithium, thanks to its small size, prepares the way for europium intercalation by pre-spreading the graphene planes. A chemical jack of sorts..
This work has now been transposed to intercalation in BCN-type structures, similar to graphite. By partially modifying the host material, one of the objectives will be to obtain compounds with interesting superconductivity and/or magnetic properties. Electronic structure calculations suggest that a high lithium content, well above that of LiC6, could be achieved, leading to electrode materials with interesting electrochemical performance
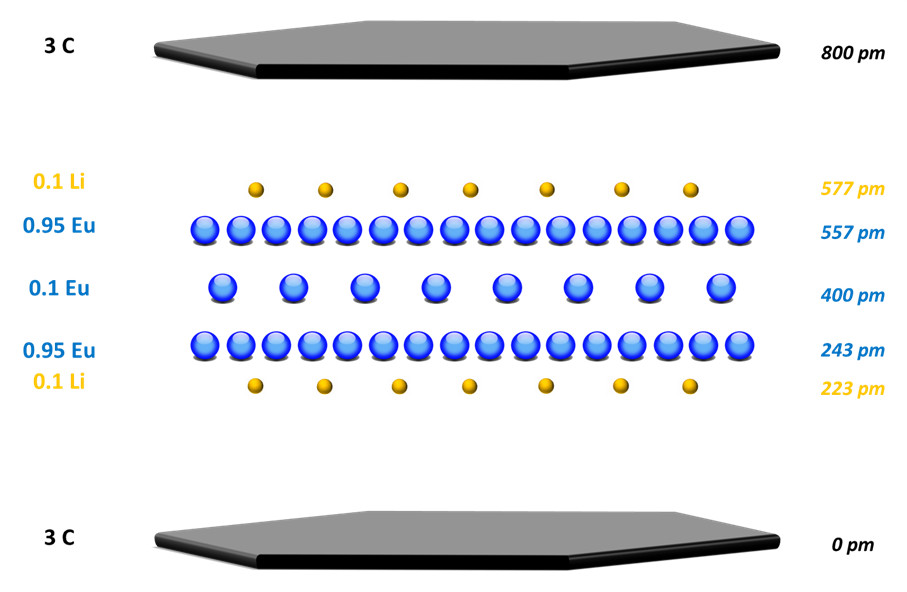
References:
A. Hérold Bull. Soc. Chim. Fr. 187 (1955) 999.
S. Cahen, H. Rida, P. Berger, M. Fauchard, P. Lagrange, J.-F. Marêché, C. Hérold, Carbon, 77 (2014) 803.
CEA-IRAMIS contact: Pascal Berger (NIMBE/LEEL)
Collaboration :
-
C. Herold, S. Cahen, H. Rida, M. Fauchard, P. Lagrange, J.-F. Marêché,
Chemistry and Physics of Solids and Surfaces Department (CP2S),
Institut Jean Lamour, UMR 7198 CNRS-Université de Lorraine, B.P. 70239, 54506 Vandœuvre-lès-Nancy Cedex, France. - P. Berger, Laboratoire d’Etude des Eléments Légers (LEEL), IRAMIS/NIMBE – UMR 3685 , CEA Saclay, France.