One of the goals in physical chemistry is to follow reaction paths in details. Many techniques have been developed with studies conducted in cells, effusive or supersonic beams, using laser spectroscopy, mass spectrometry etc. as investigation tools.
An original technique called “Cluster Isolated Chemical Reaction” (CICR) has been developed by the group “Dynamique Réactionnelle” of LIDyL/LFP for spectroscopic and reaction dynamics studies [1]. It allows determining the stoichiometry of the observed processes on a nanoreactor consisting of a van der Waals cluster. Recently, it has been successfully applied to helium droplet, which is a quite fascinating medium joining a very low temperature (0.37 K), superfluidity and finite size. The latter allows trapping and isolating a controlled number of molecules and stabilizing complexes which have a very low stability. The study of such systems provides very accurate information on the intramolecular potential energy surfaces (PES), improving the reliability of the associated models.
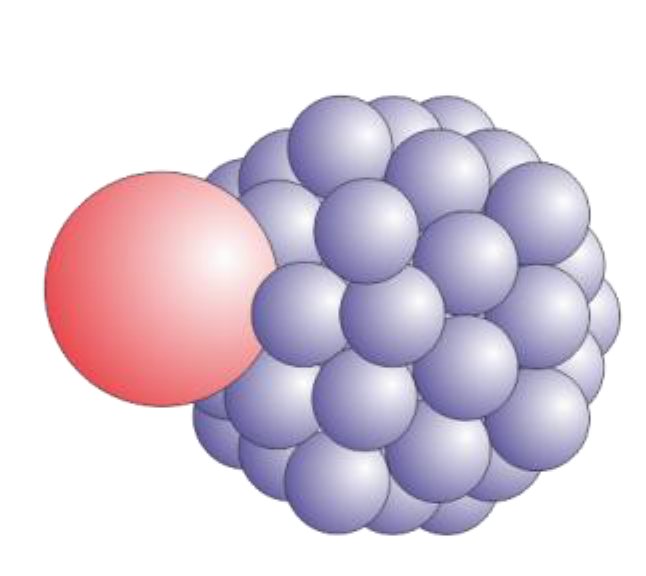
Compared to studies by molecular beam techniques, the “Cluster Isolated Chemical Reaction” CICR technique consists in studying molecules trapped by noble gases (neon, argon …) or molecular (nitrogen, methane, etc.) clusters. The low temperature of helium droplets and their superfluid nature allow forming and stabilizing molecular complexes of very low stability, difficult to obtain in the gas phase and unobservable at room temperature. The superfluid character allows studying dynamics and spectroscopy, unaffected by the viscosity of a solvent or by the rigidity of classical van der Waals cluster or matrix. For instance, high resolution ro-vibrational spectroscopy is accessible in helium droplet in contrast with other media such as rare gas matrices or even para-H2 matrices. An added benefit of the low temperature is a great simplification of the spectra, because the initial state is the lowest energy one. This is particularly advantageous when several isomers coexist (case of large organic and bio-organic molecules, sugars …), as structural information can be obtained on each isomer.
Although superfluid, a weak interaction still exists between the helium droplet and the hosted molecules which affects dynamics. This opens the door to original spectroscopic and chemical reaction dynamics studies where the hosted species interact with a quantum solvent.
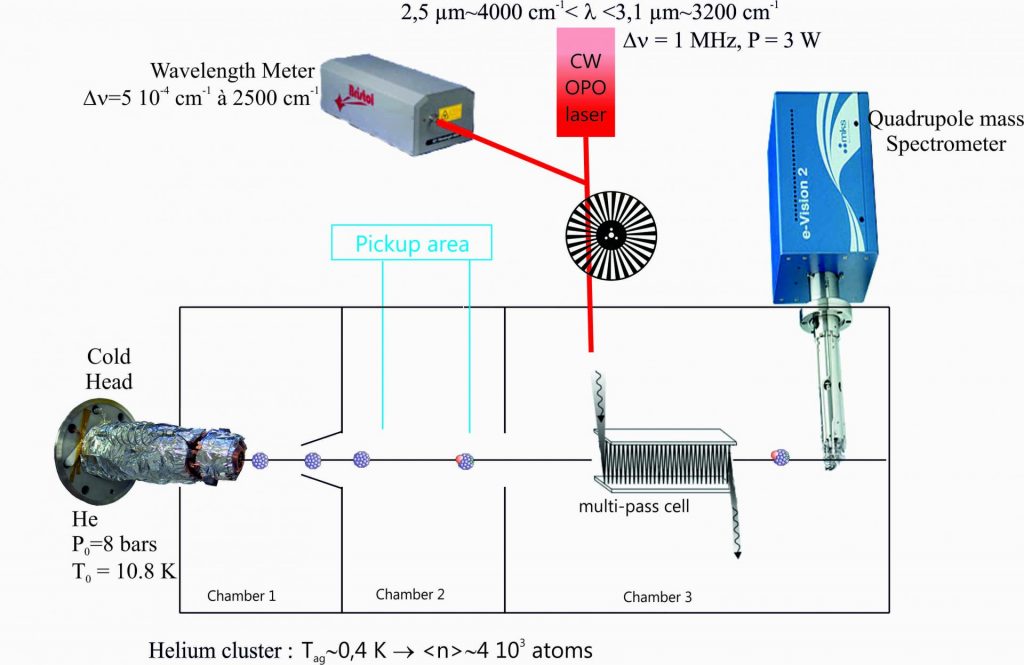
Figure 1 displays a scheme of the “GOUTTELIUM” setup. Helium clusters are generated by condensation in a supersonic beam (generating conditions: T0 = 10.8°K, P0=8 bars, D*=5 µm). Their average size is ca four thousand atoms. After extraction by a skimmer, the helium cluster beam passes through a cell containing a low acetylene pressure. These molecules are deposited on the clusters by a collisional process[1]. Downstream the pick-up cell, the cluster beam crosses an effusive beam of argon. This arrangement allows depositing a controlled amount of acetylene molecules and argon atoms on the helium droplets. These two species migrate and associate together to form a complex because their association energy, of the order of 17 meV [2] (140 cm-1 or 200 K), is much higher than the temperature of the cluster, 0.032 meV (0.37 K).
The cluster beam then enters into the analysis chamber, where the C2H2-Ar complex hosted by the droplet is excited by an infrared OPO (Optical Parametric Oscillator) laser which is tunable between 2.5 and 3.1 micrometer. Since the oscillator strength for absorption at these wavelengthes is very weak, large laser powers must be used (3 W in our case) associated with a multi-pass cell (30 reflections in our case). This increases the volume of interaction between the clusters beam and the laser beam (~50 mm3). The transmitted flux of clusters is measured by a quadrupole mass spectrometer tuned to the mass of the He2+ ion. Helium dimer are known indeed to track helium droplets upon ionization in a quadrupole mass spectrometer.
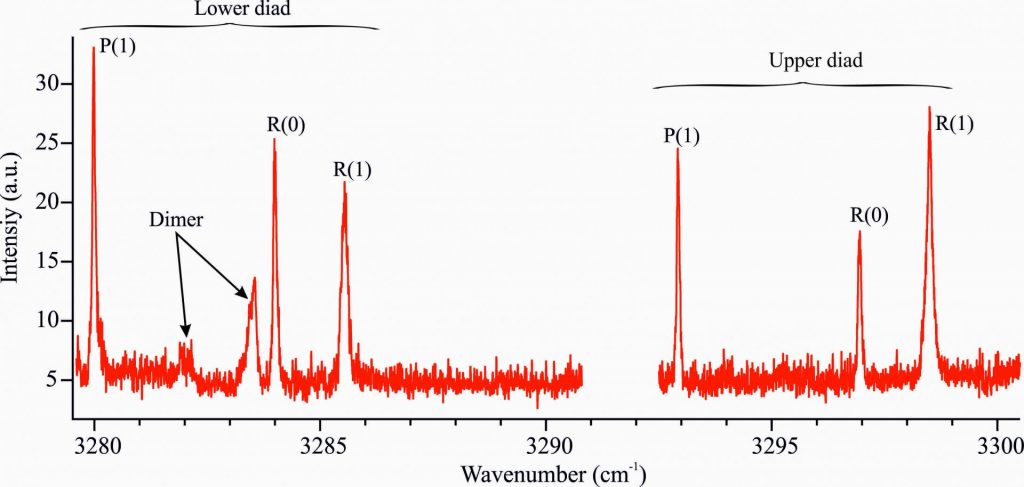
Infrared spectroscopy of doped helium clusters is based on the fact that ro-vibrational excitation of the hosted molecule (C2H2 or C2H2-Ar here) provides excess energy which is eventually relaxed into the droplet. This corresponds to several hundreds or thousands of cm-1 (1 cm-1~ 0.12 meV) compared to the binding energy of a helium atom in the droplet (5 cm-1 = 0.62 meV) [3]. Hence, several hundreds of helium atoms are lost by the droplet upon the absorption of a photon. During the interaction time, the solvated molecule has time to make several absorption-relaxation cycles, thus causing a full evaporation of the cluster. A significant reduction of the cluster flow is observed when the laser is tuned to a molecular transition of the solvated molecule. An experiments consists in recording the transmitted flow of clusters as a function of the laser wavelength.
Figure 2 shows the first infrared absorption spectrum of acetylene obtained with the GOUTTELIUM setup. It is composed of two sets of ro-vibrational lines (P(1), R(0) and R(1)) called a diad, which show a coupling (Fermi resonance) between a normal mode of vibration (ν3) and a combination of normal modes of vibration ((ν2+ν4+ν5).
Our current work consists in understanding the evolution of this type of resonance when a perturbation of the vibrational states of the acetylene molecule exists. The use of helium clusters allows forming complexes acetylene-argon with a perfectly controlled number of argon atoms. Thanks to the CICR technique it is possible to determine the stoichiometry of the complex associated to each absorption line. This complex, without real chemical bond, is called “soft” and the movements of the intramolecular vibration are strongly anharmonic. This allows getting detailed information on the potential energy surface, as well as on the interactions between the internal vibrationally excited states of the molecule and the van der Waals modes (with argon).
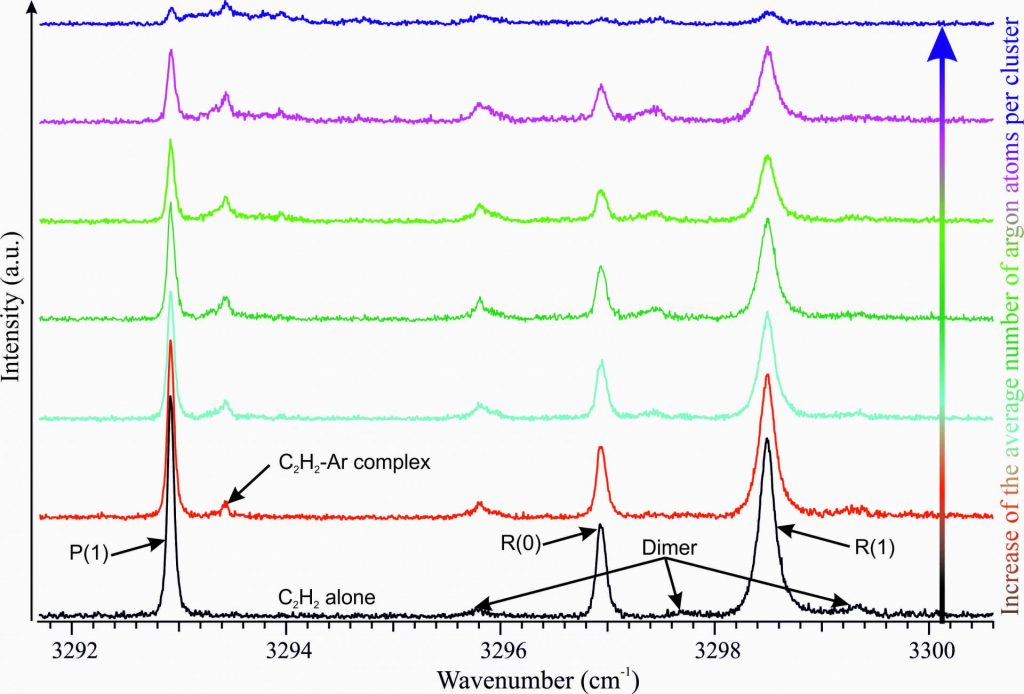
The figure above shows the first infrared absorption spectra of the complex acetylene-argon obtained on the GOUTTELIUM setup. In this figure, the number of argon atoms vary from 0 (bottom spectrum) to 3.5 (top spectrum) in average. The analysis of the spectra should allow understanding the evolution of various molecular bands and assigning those from complexes with 1, 2 or more argon atoms. We thus observe the evolution of the Fermi resonance in the presence of more and more perturbers.
As shown in the study of the complex acetylene-argon, the new apparatus “Gouttelium” allows realizing a very fine spectroscopy of molecular complexes of very low stability. The high quality ro-vibrational spectra, as obtained at very low temperature, provide detailed data on intramolecular potential, essential to obtain reliable modeling of molecular potential energy surfaces to describe the detailed mechanisms of elementary chemical reactions.
A more complet study of van der Waals complexes of acetylene- rare gas (Ne, Ar, Kr) , can be found in [4] (see figure 4) and [5].
References :
[1].Cluster isolated chemical reactions, J. M. Mestdagh, M. A. Gaveau, C. Gee, O. Sublemontier, J. P. Visticot, International Reviews In Physical Chemistry 16, 215 (1997).
[2].The intermolecular potential of Ar-acetylene. Information from infrared and microwave spectroscopy, A. E. Thornley, J. M. Hutson, Chemical Physics Letters 198, 1 (1992).
[3].Successive capture and coagulation of atoms and molecules to small clusters in large liquid helium clusters, M. Lewerenz, B. Schilling, J. P. Toennies, Journal of Chemical Physics 102, 8191 (1995).
[4].Large Amplitude Motion within Acetylene-Rare Gas Complexes Hosted in Helium Droplets, M. Briant, A. Viel, E. Mengesha, M.-A. Gaveau, B. Soep, J.-M. Mestdagh, P. Jamet, J.-M. Launay, L. Poisson, Physical Chemistry Chemical Physics 21, 1038 (2019).
[5].Large amplitude motion of the acetylene molecule within acetylene-neon complexes hosted in helium droplets, M. Briant, E. Mengesha, P. de Pujo, M. A. Gaveau, B. Soep, J. M. Mestdagh, L. Poisson, Physical Chemistry Chemical Physics 18, 16414 (2016).
CEA-LIDyL-LFP Contact : Marc Briant.
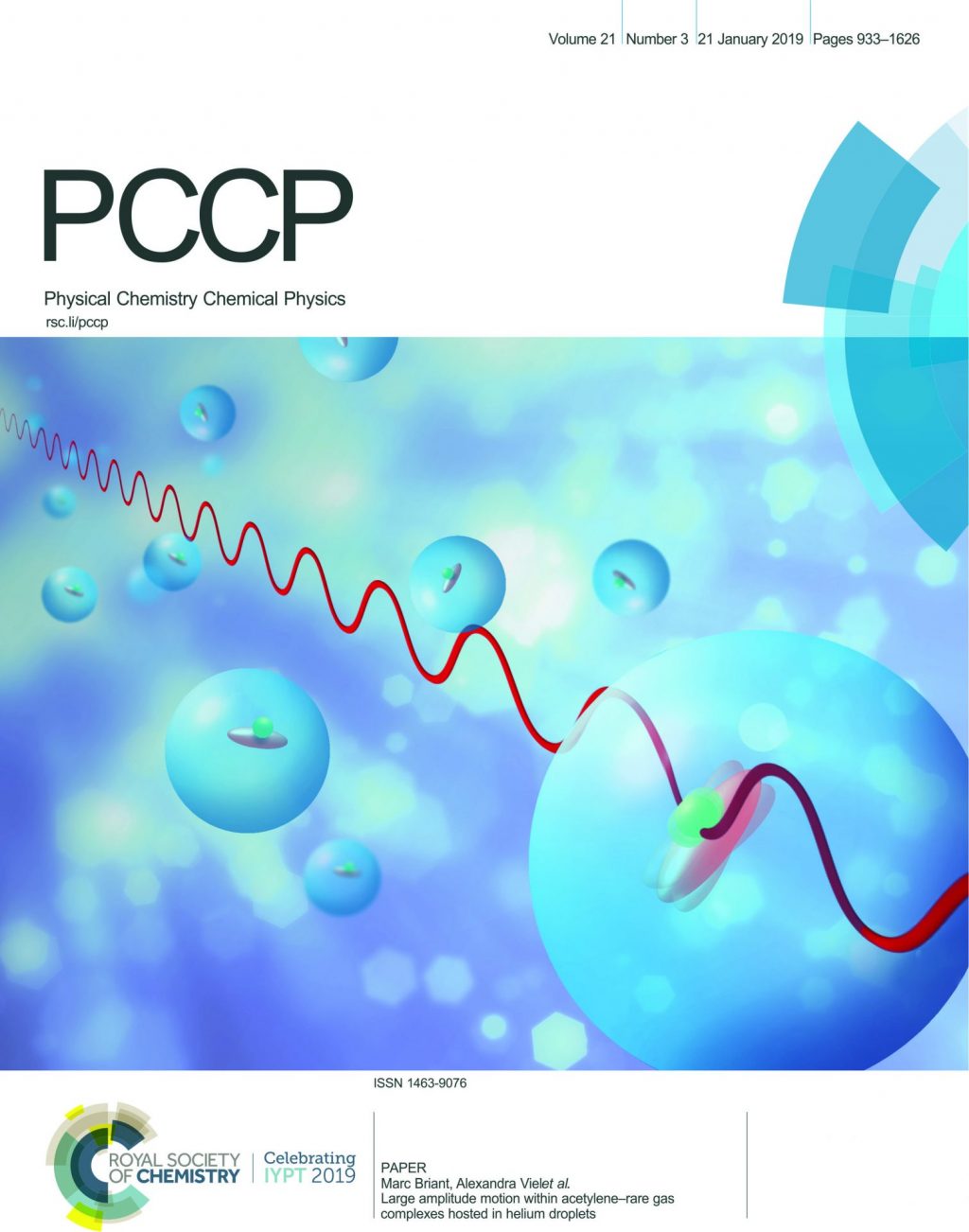