W. Boutu1, S. Haessler1, H. Merdji1, P. Breger1, G. Waters2, M. Stankiewicz3, L. J. Frasinski4, R. Taieb5,6, J. Caillat5,6, A. Maquet5,6, P. Monchicourt1, B. Carre1 and P. Salieres1
2. J.J. Thomson Physical Laboratory, University of Reading, Whiteknights, Reading RG6 6AF, UK
3. Institute of Physics, Jagiellonian University, ul. Reymonta 4, 30-059 Kraków, Poland
4. The Blackett Laboratory, Imperial College London, Prince Consort Road, London SW7 2BW, UK
5. UPMC Univ Paris VI, UMR 7614, Lab. de Chimie Physique-Matière et Rayonnement, 11 rue P. et M. Curie, 75231 Paris Cedex 05, France
6. CNRS, UMR 7614, LCPMR F-75005 Paris, France
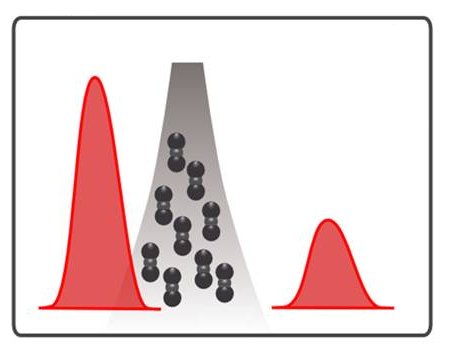
Today lasers cover a wide range of wavelength and pulsed lasers provide a temporal resolution that allows for example to observe the stages of a chemical reaction. Ultra-short pulse lasers now reach the range of hundred of attosecond, the attosecond (1 as = 10-18 s) being the characteristic time associated with the movement of electrons in atoms. Active research in this area is carried on with a high competition to produce laser light with increasingly high frequency (X-ray range), and shorter pulse duration. In this field, the generation of high order harmonics in an atomic gas has already demonstrated its potential [1]). New experiments of harmonic generation in a molecular gas show that it becomes possible to control and shape the “as” laser pulses.
When an intense pulse of infrared laser light is focused on an atomic beam, it induces ultra-fast electronic dynamics on a sub-femtosecond time scale. The resulting emission of short light pulses covers a wide spectral range up to the X-ray range. The duration of these pulses can reach ~ 0.1 femtosecond (= 100 attosecond, measured at SPAM in 2003), which makes them the shortest pulses ever made.
However, the possibilities of controlling the pulses generated by atoms remained limited, restricting the scope of applications. We have shown that the generation in small diatomic molecules such as N2, O2 or linear as CO2, allows an effective control of the attosecond emission: the presence of several atoms perturbs the intramolecular electronic dynamics and leads to quantum interferences modulating the attosecond emission. Such interferences strongly depend on the angle between the axis of the molecule and the electric field of the laser light. By controlling the alignment of molecules with a weak first laser pulse, it becomes possible to “shape” the emitted attosecond pulses. Such pulses could help in the future to control coherently ultrafast atomic and molecular processes.
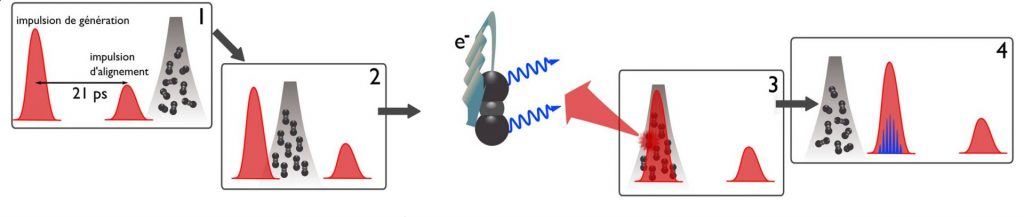
A first laser pulse (50 fs, intensity ~ 5 x 1013 W/cm²), focused on a molecular (CO2) beam, aligns the molecules in the direction of the laser field by coupling of the induced dipole with the field (Fig . 1.1 and 1.2). A second laser pulse (21 ps later) of same wavelength but higher intensity (~ 1014 W/cm²), and whose direction of polarization is variable, generates the attosecond pulses (Fig. 1.3): the laser field “frees” by tunnel ionization an electronic wavepacket that is accelerated and returns to the ion where it recombines radiatively, converting its kinetic energy into a pulse of extreme UV radiation (XUV). In a molecule, recombination may occur on each atom, but the respective emissions are dephased due to the path difference between the electronic wave packet trajectories.
When this difference becomes comparable to the half-wavelength of the electronic wavepacket, a destructive interference modulates the temporal profile of the attosecond emission (Fig. 1-4), and thus distorts its amplitude and spectral phase. By measuring these two quantities, it was possible to observe without ambiguity for the first time the intramolecular quantum interference. Moreover, by changing the direction of alignment of the molecules with respect to the polarization of the generating laser field, the difference in “electronic path” and thus the relative phase of the intramolecular emissions are finely modified: in that way, one can precisely control the interference process and manipulate the attosecond pulses (duration and synchronization with the laser field).
Beyond this demonstration of a control of the XUV emission on the attosecond timescale, this study marks a milestone towards ultra-fast imaging of molecular orbitals. Indeed, these measurements give access to the molecular dipoles governing the recombination for different orientations, and in principle would allow a tomographic reconstruction of the radiating orbital.
These studies were performed on the laser LUCA of IRAMIS, by an England-Poland-France collaboration involving 5 laboratories. The 2 french laboratories (IRAMIS/SPAM and LCPMR of University of Paris 6) are partners in the Atto-science ANR programme.
Références :
[1] Attosecond Synchronization of High-Harmonic Soft X-rays,
Y. Mairesse, A. de Bohan, L. J. Frasinski, H. Merdji, L. C. Dinu, P. Monchicourt, P. Breger, M. Kovacev, R. Taïeb, B. Carré, H. G. Muller, P. Agostini, and P. Salières, Science 302 (2003) 1540.
Coherent control of attosecond emission from aligned molecules W. Boutu, S. Haessler, H. Merdji, P. Breger, G. Waters, M. Stankiewicz, L. Frasinski, R. Taieb, J. Caillat, A. Maquet, P. Monchicourt, B. Carré and P. Salières, Nature Physics 4 (2008) 545. |
CEA-CNRS press release (in french).
Optics & Photonics Focus highlight: “Towards Filming Chemical Reactions” by Armand Niederberger, (version PDF)
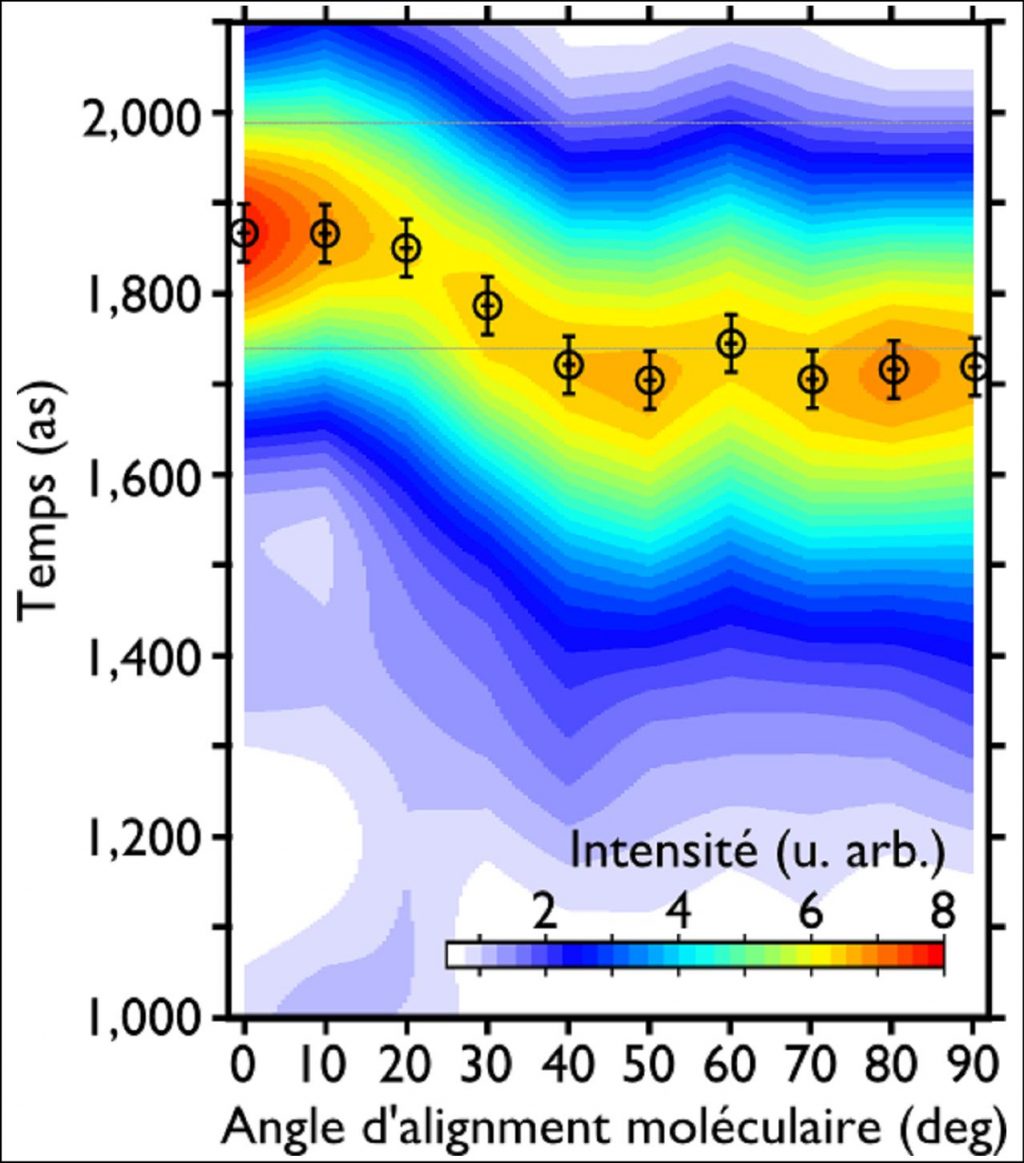
The ability to amplify such pulses (on a femtosecond timescale) by a free electron laser device broadens the field of their applications (see IRAMIS highlight: “Coherent harmonics of a Free Electron Laser radiation from gas generated harmonics“.