Liquid Electrolyte based Multivalent Batteries
The development of lithium-ion batteries has played a key role in this development of intermittent renewable energies and mobile technologies. However, this technology seems to be reaching its limits and new solutions are emerging, such as magnesium batteries. Magnesium metal is an attractive negative electrode because of its abundance and its high volumetric and gravimetric capacities combined with a quite low redox potential. The divalent nature of Mg2+ offers two electrons per metal compared with just one in the case of alkali metals such as Li and Na.
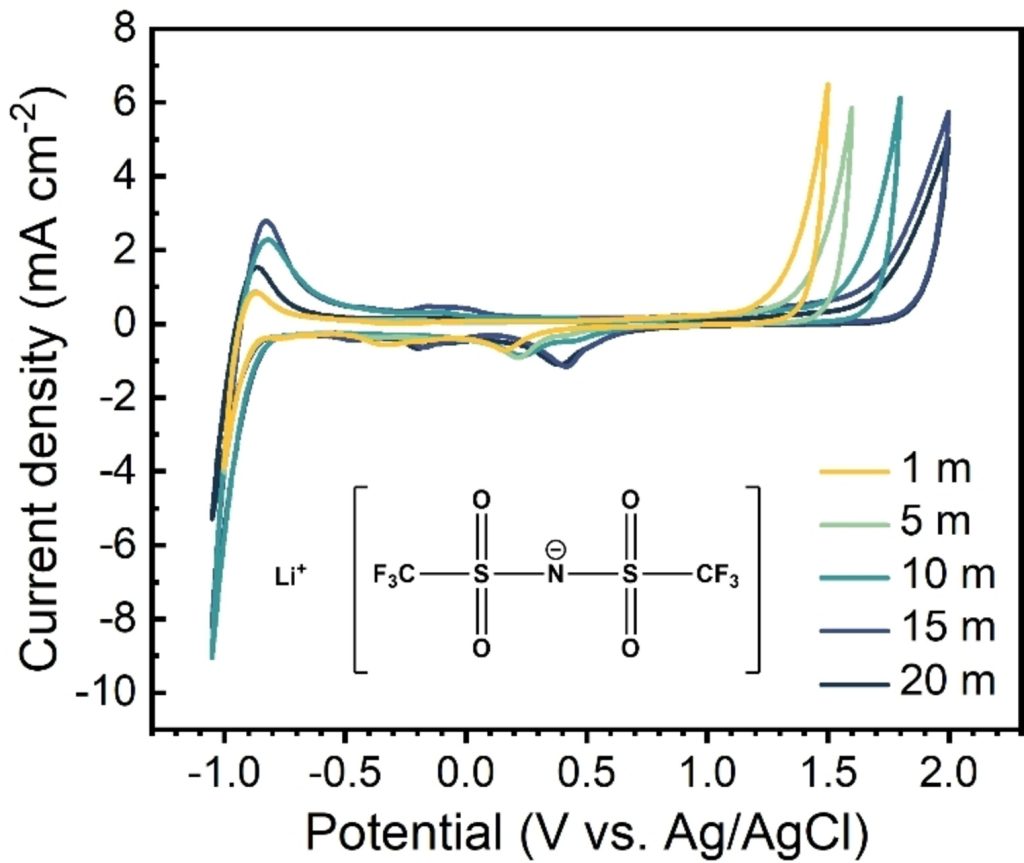
The operation of a Mg battery is similar to that of a Li-ion battery, involving the exchange of Mg2+ ions between a positive electrode and a negative electrode, ideally magnesium metal, via an electrolyte solution. The main obstacle to making magnesium batteries is the lack of suitable electrolytes with a wide potential window that we can combined with magnesium metal or effective negative electrodes.
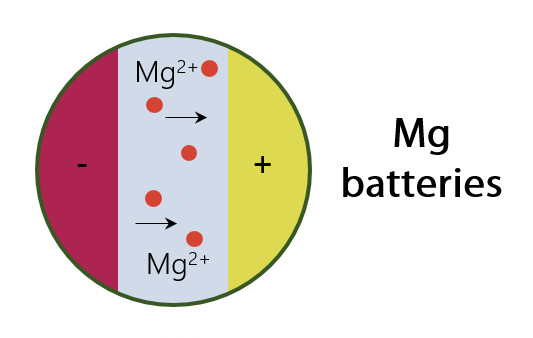
At LEEL, we are tackling these challenges with two different approaches. One of the solutions is to replace the Mg metal at the negative electrode with compounds that form an alloy with the Mg and are stable to conventional electrolytes. This has been investigated within the MAGICIEN ANR project (2017-2021). Our second approach is to focus on the development of new electrolytes, particularly with the concept of concentrated aqueous electrolytes for Mg-ion batteries, in the frame of the MAGWAT ANR project.
Solid Electrolytes for Lithium and Sodium Batteries
Solid-state batteries are considered as a next-generation battery technology with many potential improvements over the current state-of-the-art Li-ion in terms of safety, power, and energy density. The development of efficient, high ionic conductive solid electrolytes is crucial for the progress in solid-state batteries. Low-cost synthesis and their optimization with different battery electrodes is seen as a decisive factor. Towards this direction, researchers at LEEL work on two important advanced electrolyte categories: ceramic and polymer-ceramic composite electrolytes.
Ceramic solid electrolytes: Our group has been active in developing new solid electrolytes for lithium and sodium solid-state batteries. We concentrate on the synthesis, structural characterization, and battery application studies of Garnet, Nasicon, and Halide solid electrolytes.
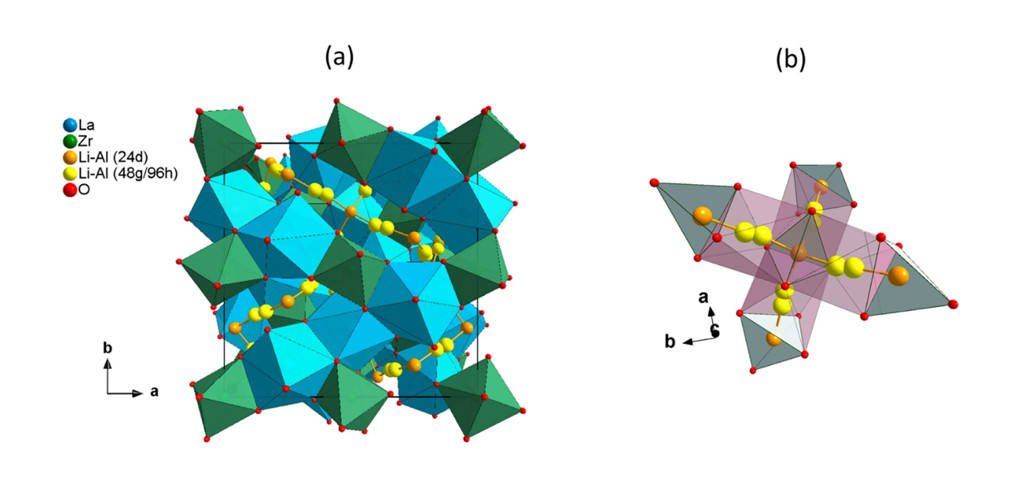
Halide ionic conductors are recently revisited and applied in advanced solid-state batteries. We aim to understand the effect of synthesis conditions, doping and lithium concentrations on the ionic motion (diffudion) within the structure and the ionic conductivity. In addition, a major focus of the research is to employ this newly developed electrolytes in various electrode composites (NMC, LFP, Sulfur and Silicon) and study their electrochemical performaces.
The electrode kinetics of solid electrolytes play a crucial role in various solid-state electrochemical applications including batteries. The understanding of the diffusion phenomena in ceramic electrolytes with the help of high-frequency impedance measurement coupled with detailed structural studies with XRD and NMR constitutes the major activity of the group. In other words, we try to understand the movement of ions in the electrolyte and electrode, and how we can influence their activation energy barrier and stability by carefully modifying the structure by doping or by changing the synthesis conditions.
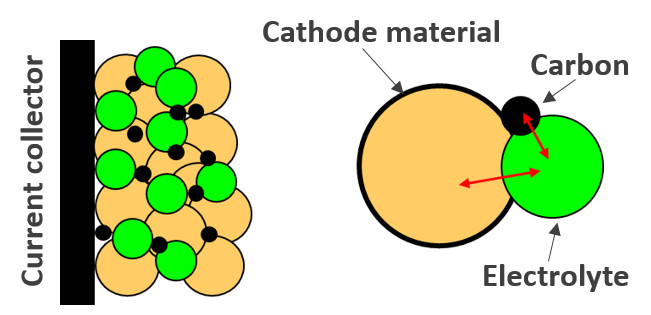
Polymer-ceramic composite electrolytes: Polymer electrolytes enable the application of lithium metal anodes in solid-state batteries, potentially hindering the dendrite growth and short circuits. They also enable enhanced safety, processability, adhesion to the electrodes, and flexibility. The currently used PEO-based polymer electrolytes require a high working temperature in the range of 60-80 °C for their nominal performance. Our research focuses on the inclusion of oxide and nasicon ceramic solid electrolytes in the polymer matrix to improve their ionic conductivity and reduce the operating temperature. Besides a further understanding of the charge transport mechanism in these electrolyte systems and the processes taking place at the interface with the metallic anode with EIS and solid-state NMR studies, our work particularly focused on increasing the ceramic content in the polymer matrix.