Lithium-oxygen (Li-O2) or lithium-air batteries are possible alternatives to lithium-ion batteries for energy storage. They offer a high specific energy of ~ 3500 Wh kg-1 that is more than ten times higher than current Li-ion batteries. However, Li-O2 batteries are far from mature and have not yet reached their full potential, mainly due to their limited number of charge-discharge cycles. More knowledge about the operation of this type of batteries is needed to improve their performance.
Operando studies, where analytical data are acquired on an operating battery, are essential to obtain a clear view of the reaction mechanisms involved. However, it is a challenge to adapt these techniques to a metal-oxygen battery, since it requires a continuous supply of oxygen. A team from IRAMIS designed a cell with an oxygen gas tank, allowing the operation of a Li-O2 cell inside a magnet of a nuclear magnetic resonance (NMR) spectrometer during the acquisition of spectra. The results allow to detail the electrochemical and degradation mechanisms within an operational Li-O2 battery.
Metal-oxygen (M-O2) batteries have attracted attention in recent years because they offer the highest energy density. Among them, the lithium-oxygen battery theoretically offers a specific energy of ~ 3500 Wh kg-1 in the discharged state, with the combination as active materials of lithium, a light metal, and oxygen, naturally abundant in the atmosphere.
During the discharge of a Li-O2 battery, an electrochemical reaction between Li+ ions and oxygen O2 leads to the formation of lithium peroxide Li2O2. However, the performance of Li-O2 batteries remains rather poor, due to parasitic chemical reactions during charging, involving the electrolyte solutions, singlet oxygen, Li2O2 and carbon electrodes and leading to the formation of the compounds: CO2, Li2CO3, lithium-formate (CHLiO2) and -acetate (CH3COOLi). In situ studies have provided valuable initial information on the real-time chemistry of Li-O2 batteries [1].
Nuclear magnetic resonance (NMR) can provide critical information on the mechanisms involved, particularly on the formation and behavior of amorphous products. Until now it has only been applied ex situ, after operating and the dismantling of the cell. These measurements do not give a realistic view of the operating cell and can lead to biased data and erroneous interpretations, in particular because of the high reactivity of the products formed in Li-O2 batteries, such as singlet oxygen and peroxide. The first developments of operando NMR were mainly applied to metal or metal-ion batteries based on the intercalation or insertion of the charge carrier ion. This had not yet been realized for Li-O2 batteries, due to the difficulty of incorporating an O2 gas electrode in the NMR apparatus.
The NIMBE/IRAMIS team has succeeded in designing a closed system (see figure below) with a cylindrical cell including two compartments, the first being an oxygen reservoir and the second an electrode stack. The first advantage of this closed system is that it can be easily manipulated during NMR experiments, without connection to a gas supply. Another important advantage of this setup, for the type of battery to be studied, is the absence of evaporation of the electrolyte by the gas flow. In the design of the cell, other requirements were also taken into account to allow NMR measurements:
- cell geometry adapted to the space available inside the NMR probe head;
- absence of metallic materials to avoid any interaction with the strong magnetic field;
- robust sealing and compression of the cell for cyclability and durability of the battery;
- sufficient mass of electrode material for enhanced measurement sensitivity.
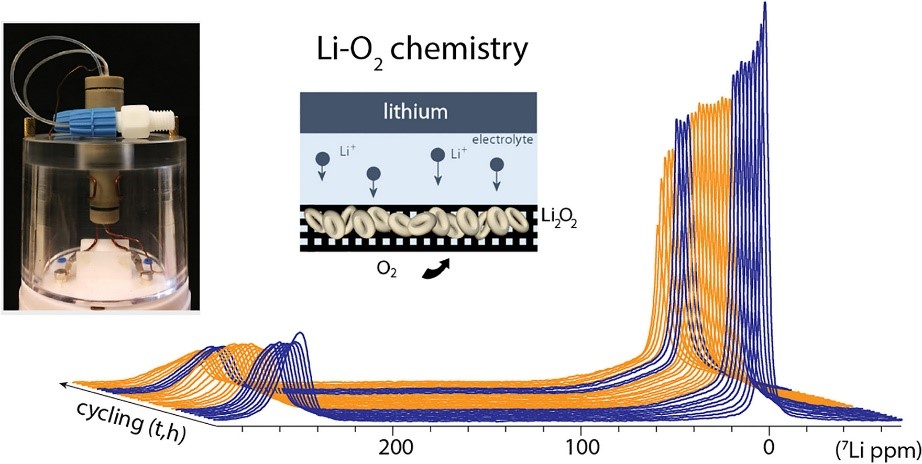