Introduction
The aim of the “Theory and simulation” activity is to develop theoretical and numerical tools for interpreting attosecond science experiments.
To this end, we are developing numerical codes to model the non-linear response of an atomic gas to an intense laser field, at both the spatio-temporal scales of the problem.
At the microscopic scale, the laser-atom interaction is described in all its generality by the time-dependent Schrödinger equation, or, on an ad hoc basis, by an experimentally measured nonlinear susceptibility.
On the macroscopic scale, processes are described by the wave equation, with the microscopic response as the source term. Different interaction geometries (jet, cell or capillary) are modeled.
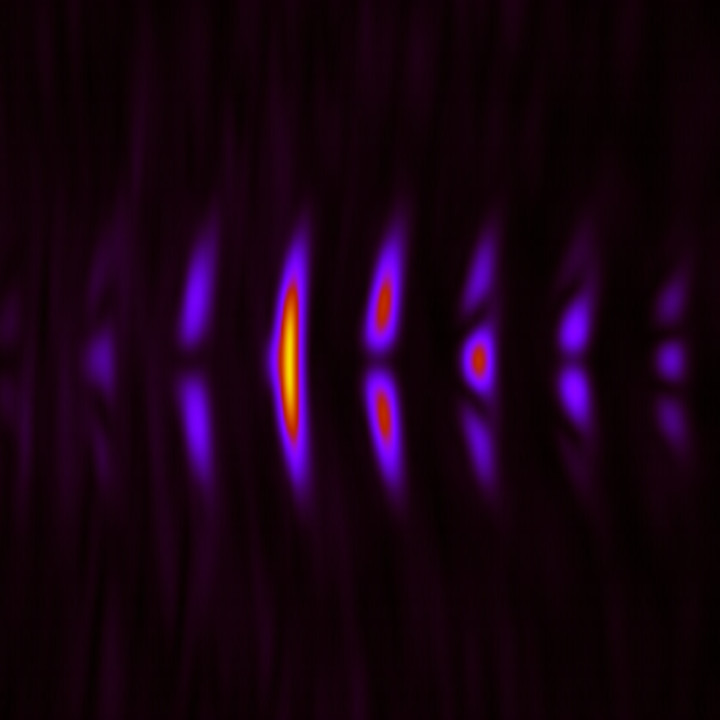
Harmonic generation by two non-colinear laser beams
We have a number of models describing harmonic generation in gases, on both atomic and macroscopic scales.
In particular, the Schrödinger equation can be solved either numerically or analytically in the Strong Field Approximation (SFA).
Opposite, an example of the modeling of a harmonic generation experiment using two non-colinear laser beams. The interference of the beams produces a transient amplitude and phase grating, leading to the observation of far-field harmonic diffraction peaks.
Read more
Figure 1 a) Far-field diffraction of harmonics 15 to 21. As the illumination of the cladding beam increases relative to that of the generator beam, a ratio noted α, higher and higher diffraction orders appear.
Figure 1 b) Evolution of the diffraction pattern with α for H15. Each diffraction order corresponds to an optimum value of α.
Figure 1 c) Position of αmax as a function of diffraction order.
Figure 1 d) Distribution in α of the different diffraction peaks.
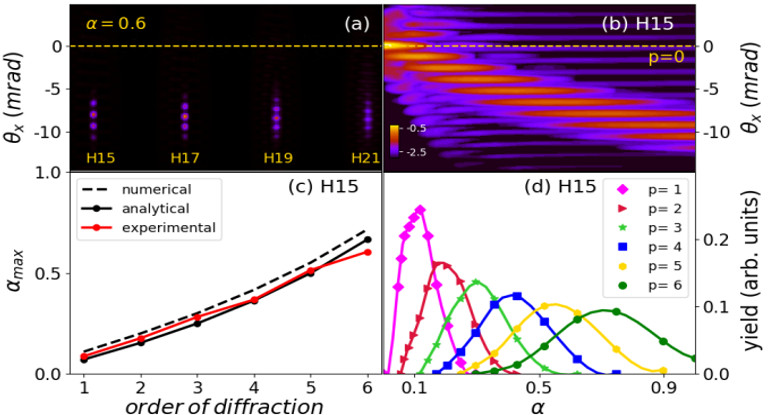
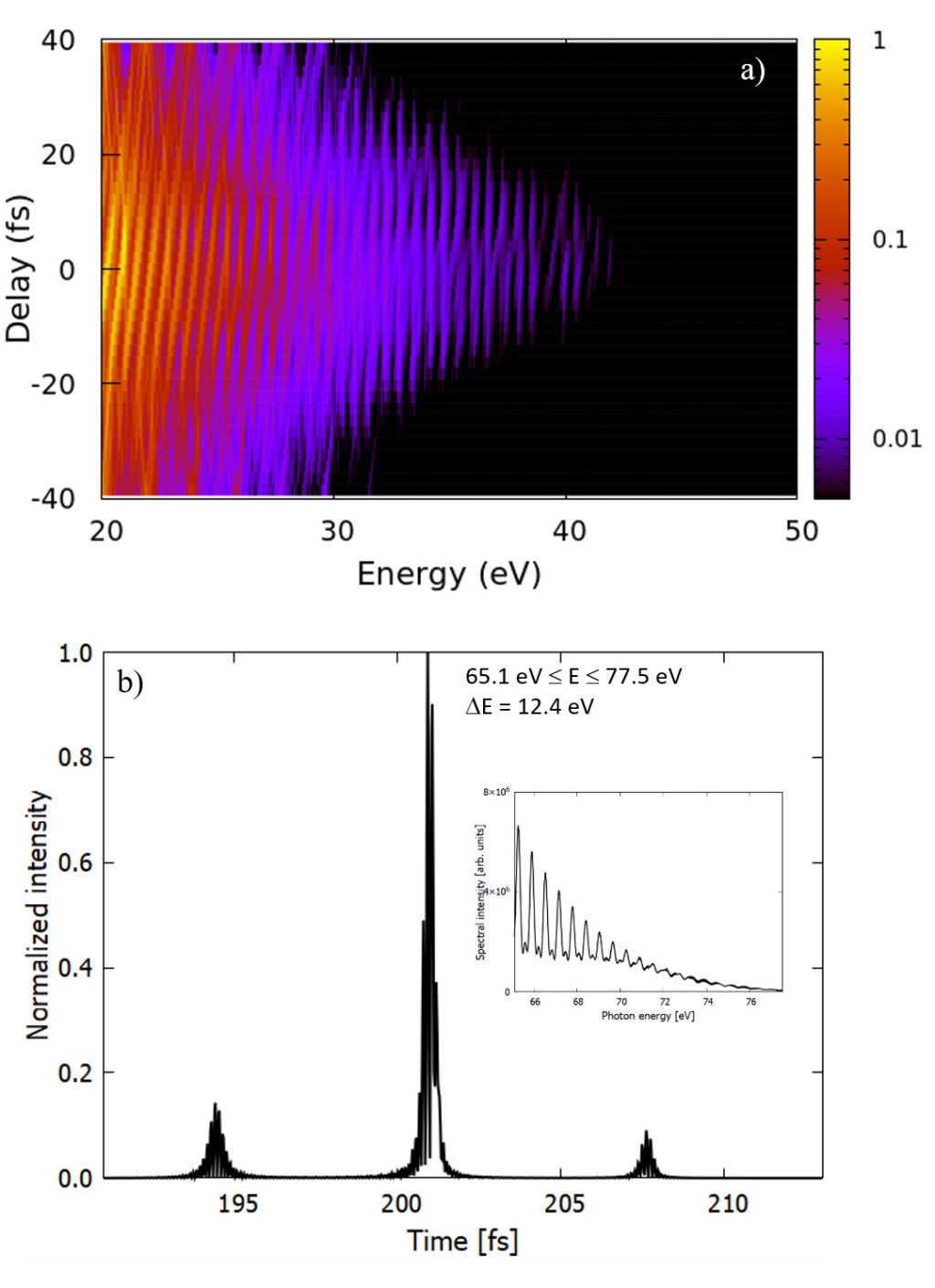
Isolated attosecond pulses by frequency mixing
An important step in attophysics is to produce isolated attosecond pulses.
To this end, two approaches are being studied in the group.
The first is to use mixing at two incommensurable frequencies to generate harmonic radiation. By blocking the periodic recombination of the electron with its parent ion, the number of emission peaks is reduced and, under certain conditions, only one peak remains.
The upper figure illustrates the evolution of the spectrum with the delay between the two pulses for wavelengths of 800 and 1300 nm.
The lower figure shows that, at zero delay, selecting a spectral band near the cutoff produces virtually a single attosecond pulse.
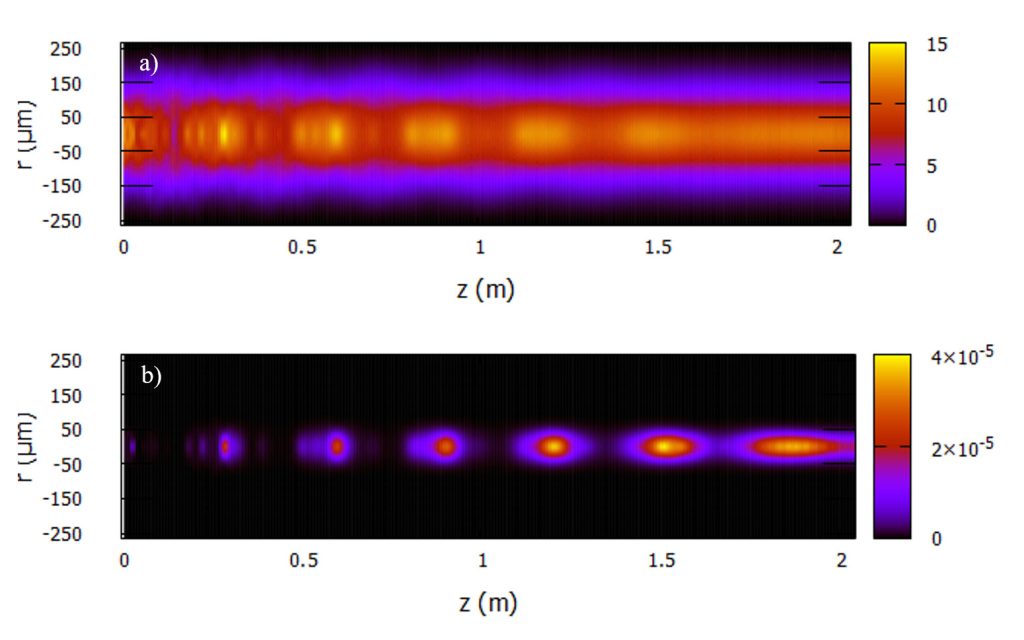
Hollow-fiber laser post-compression
The second approach to producing single attosecond pulses is to use single-cycle optical generation fields, post-compressing lasers with a duration of around twenty femtoseconds.
The post-compression, performed by “chirped” mirrors, is carried out after spectral broadening in a gas-filled capillary or multi-pass cell. Numerical activity in this type of experiment, carried out in collaboration with the SLIC group, consists in calculating spectral broadening, mainly due to the Kerr effect.
We are developing numerical models describing laser propagation, taking account of spatio-temporal couplings with the gas, for the different devices used.
Read more
By way of example, the figure opposite describes the propagation of a Ti:Sapphire laser in a capillary.
Figure a) shows the fluence, with its modulations due to modal coupling, which attenuate as the laser propagates.
Figure b) shows the final degree of gas ionization.
Laser post-compression by multi-pass cell
The transition from Ti:Sapphire lasers to Ytterbium lasers, currently underway in the laboratory, requires the use of multi-pass cells to post-compress the Ytterbium lasers, from a few hundred femtoseconds of initial duration to around 20 fs.
The figure below shows an example of the numerical results obtained for this configuration.
a) shows the broadened spectrum after 20 passes through the focus mirrors
b) shows the post-compressed pulse. The evolution of the spectral width and resulting pulse duration (Fourier transform-limited) with gas pressure are plotted in figures c) and d) respectively.
Agreement with experimental results, represented by red squares, is excellent.
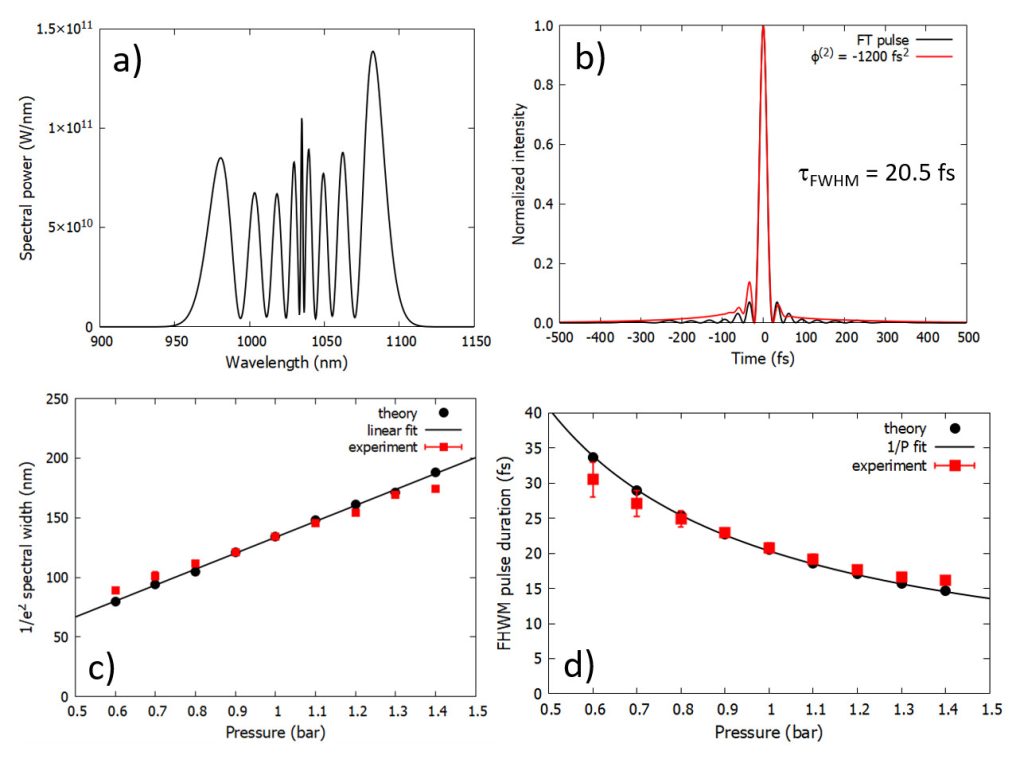
Double laser post-compression to the optical cycle
To produce a single-cycle pulse, a second post-compression stage is required under our conditions.
This can be either a second multi-pass cell or a capillary. The second option has been chosen in the laboratory.
The figure opposite shows preliminary calculations for post-compression of Ytterbium laser pulses using a capillary.
Read more
The injected pulse is post-compressed after several passes through a cell (see figure above). Figures a) and b) show, respectively, how the spectrum and pulse change with propagation.
Figures c) and d) show the spectrum at the capillary outlet and the time profile obtained, respectively. The predicted duration is close to one period of the laser field.
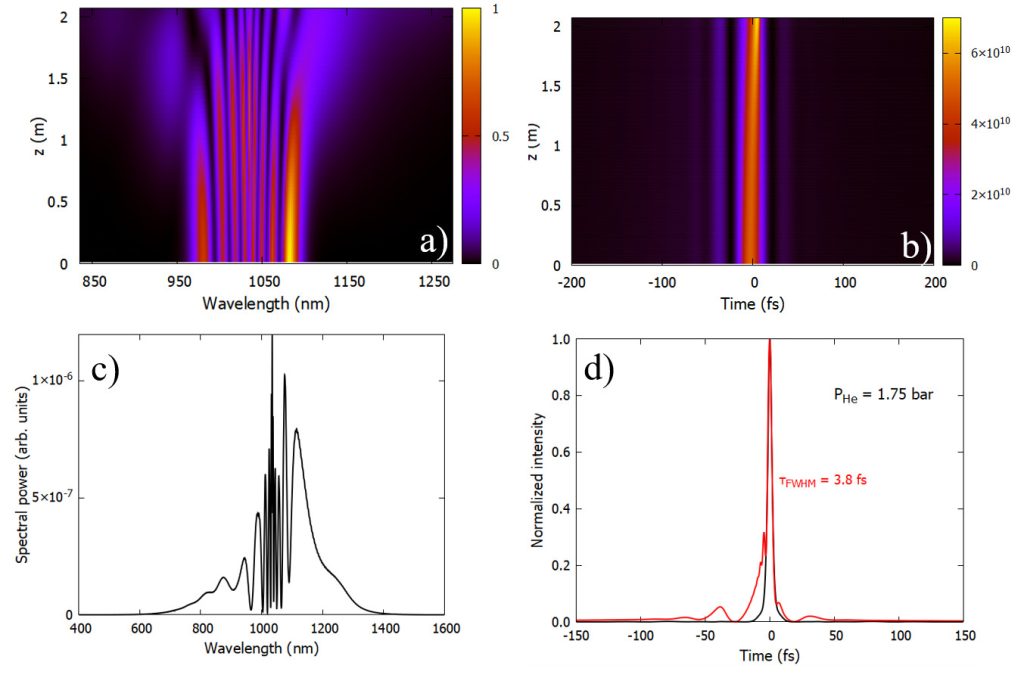