Molecular multiple ionization is easily detected with femtosecond pulses in the 1014-1015 Wcm-2 illumination range.
Small molecular ions with a charge greater than 3 are generally unstable and fragment into atomic ions, whose kinetic energy is dominated by Coulombic repulsion.
The main benefit of this fragmentation, known as the Coulomb explosion, is to image the molecular structure, provided the latter does not change during multiple ionization.
This is the case when a molecular ion accelerated to a few percent of the speed of light is stripped of its valence electrons as it passes through a sheet 30 Å thick [Science 244, 426].
The peeling time of less than 100 attoseconds “freezes” the nuclear structure of the molecule, whose evolution time is of the order of 10 femtoseconds.
This is no longer the case with visible and near-infrared laser pulses, whose optical periods and pulse durations are in the femtosecond range.
The molecule has time to stretch, and the coulombic explosion is observed at distances that differ little from one charge state to the next,
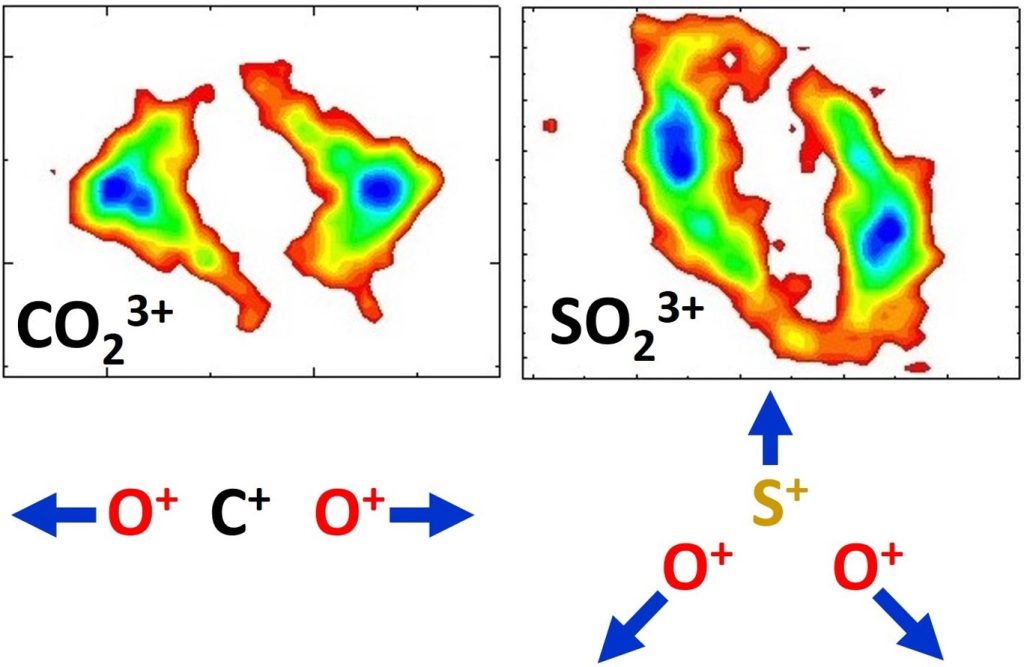
but greater than the equilibrium internuclear distances of the starting molecule.
However, the simplicity of laser excitation compared with experiments requiring a gas pedal, the recent demonstration of quasi-single-cycle laser pulses of a few femtoseconds, and the possibility of combining several pulses with attosecond temporal precision to study the dynamics of excited states make this a most promising prospect for molecular imaging.
Read more
The commonly accepted model is charge resonance enhanced ionization developed by A. Bandrauk et al. for the ionization ofH2 yielding two protons and one electron. This model has been extended to the symmetrical fragmentation of a diatomic ionA2(2q) according to the ionization schemeA2(2q-1) ®AqAq e-.
This model is more delicate for asymmetrical fragmentation, e.g. A A2 , as the asymmetrical potential seen by the departing electron significantly modifies the dynamics compared to the symmetrical case.
Our aim is to test these asymmetrical pathways in symmetrical linear molecules such as N2, O2, orCO2, with a spatially asymmetrical laser pulse obtained by coherently superimposing the fundamental frequency and the second harmonic.
Initial molecular symmetry is crucial, so as not to favor a given orientation during the initial ionization steps. Initial results show that for AA2 and A A2 ( A=N, O) fragmentation pathways in N2 and O2, the ion with the highest charge is preferentially emitted in the direction of the field asymmetry. Although this observation is consistent with an electron shift along the laser field, it does not fit well with a model of single-electron ionization from dissociative pathways such as A A or A A .
Furthermore, the field-dependent orientation of asymmetric pathways is more pronounced at low fragmentation energies, i.e. at greater internuclear distances. Taken together, these results suggest an ionization model with several active electrons and the possibility of electron recapture as a function of internuclear distance.
Experimental examples
The figure opposite shows a time-of-flight covariance map for identifying O2 fragmentation pathways, where the islands in the false-color map on the right correlate with the ions appearing in the time-of-flight spectrum on the left.
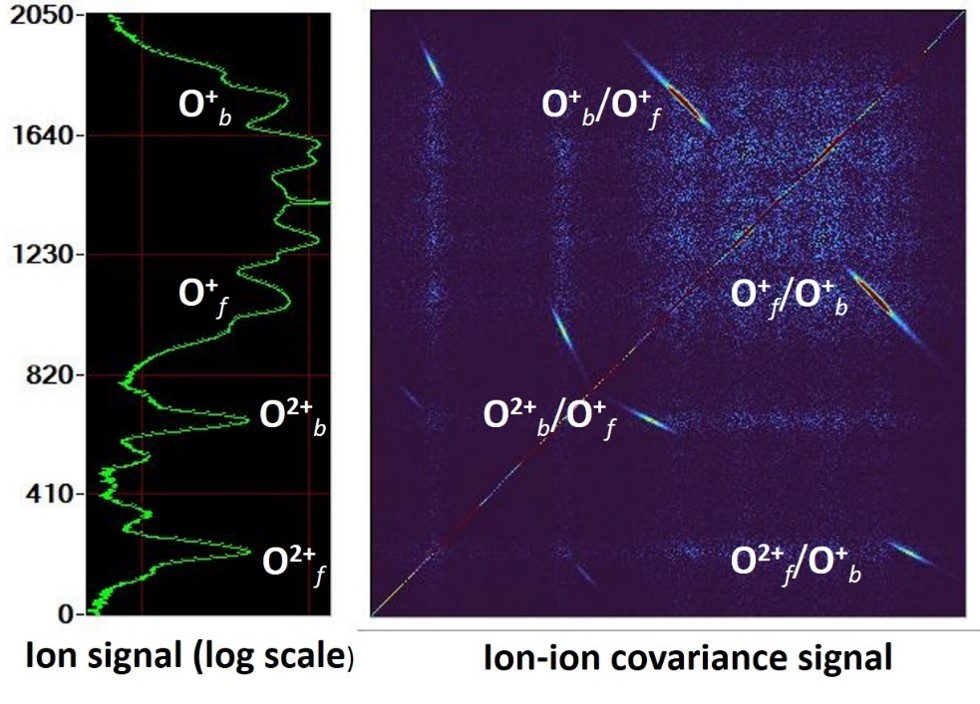
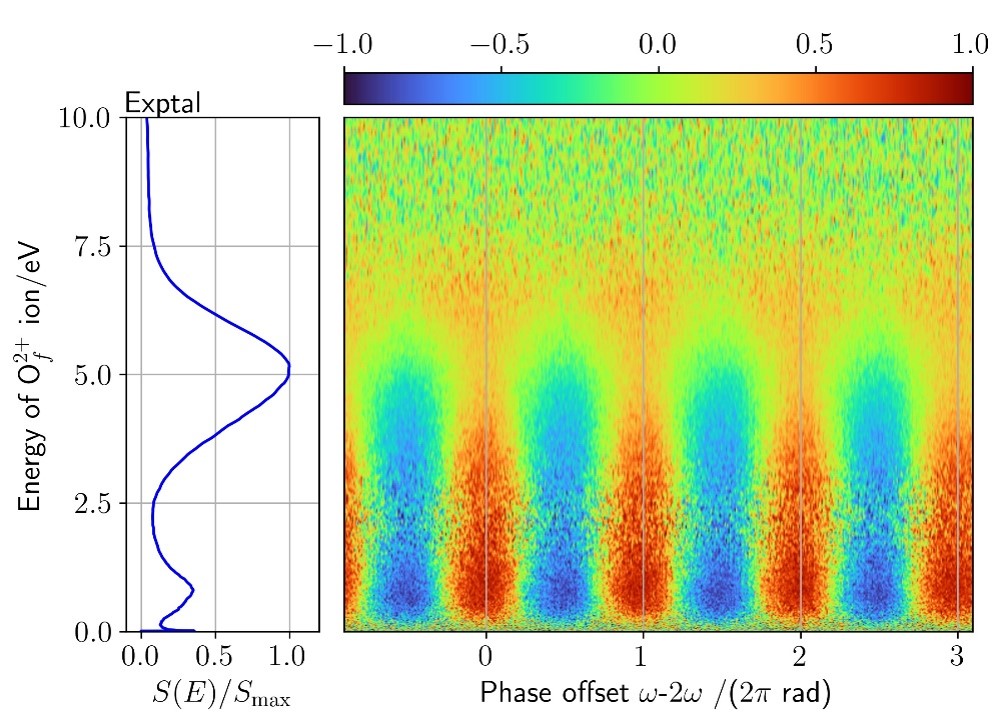
The bottom left figure shows the spectrum of O2 ionsbelonging to the O O2or O O2 pathways.
The figure above associated with this spectrum is a differential measure of the spatial orientation of these pathways as a function of the spatial asymmetry of the field.