Ultra-short pulse lasers are at the heart of research into radiation-matter interaction, with numerous applications in a wide variety of fields: femto-chemistry, photovoltaics, etc. Some of these lasers require complex developments to ensure that the pulses produced meet specific requirements in terms of their characteristics (delivered energy, wavelength, pulse shape and stability, etc.).
For ATTOLAB, the Equipex project on ultra-fast dynamics coordinated by LIDYL at the CEA, several atomic and molecular physics and solid-state physics experimental set-ups will be accessible, for which it is necessary to produce high-energy laser pulses (a few 10 mJ) at high frequencies (1 or 10 kHz) with tunable wavelength and spectral width spectra, lasting less than 20 femtoseconds (1 femtosecond = 10-15 s), with extremely precise control of the characteristics on the scale of the electric field.
In order to meet the objectives of the experiments, researchers at the “Impulse” joint laboratory between LIDYL and Amplitude Technologies have developed a laser amplifier chain with an original configuration of amplifier stages, enabling them to achieve the required characteristics at world-class levels.
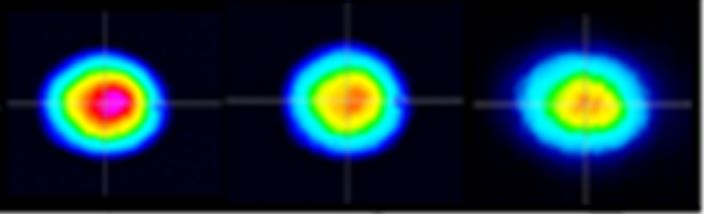
Because of the coupling between electromagnetic waves and electrical charges, lasers have become an essential tool for exploring the electronic structure of matter. Today, for example, we know how to obtain intense pulses with sub-femtosecond durations, characteristic times for electronic relaxation or photoionization. To achieve these performances, it was necessary to discover several laser techniques, such as CPA (Chirped Pulse Amplification)
Producing ultra-short laser pulses involves a chain of amplifiers, the first of which are high-gain with low energy conversion. The amplifiers in the next stage, on the other hand, have lower gain, but high energy extraction. Two main problems can then degrade the spatial and spectral quality of the beam:
- On the whole chain, as the gain is spectrally limited, amplification leads to spectral narrowing
[2] . The production of ultra-short pulses requires the widest possible spectral range. - Despite cooling by circulating water at room temperature, thermal effects induce index variations in the amplifying medium that can generate a thermal lens of very short focal length
[3]
The first amplification stage consists of a regenerative cavity that delivers a gain of106, while maintaining good spatial beam quality. However, when the cadence is increased to 10 kHz, the associated thermal focal length becomes very short (typically a few tens of cm). The cavity is then unstable and the spatial profile of the beam is degraded, as shown in the lower profiles of Fig. 1b for a cavity with a Titanium-Sapphire amplifier crystal. In this example, the pump power cannot exceed 13 W if a correct profile is to be maintained, which limits the extracted power to 1.8 W (see fig 1b, blue curve). The cavity developed by our team, in association with Amplitude Technologies within the joint Impulse laboratory, uses the same configuration as a conventional cavity, but the pump power is distributed over two amplifying media, enabling each crystal to be used at its maximum pump power (see diagram fig 1a). The power extracted then reaches 5.8 W, for 28 W of pump power, while preserving excellent beam spatial quality
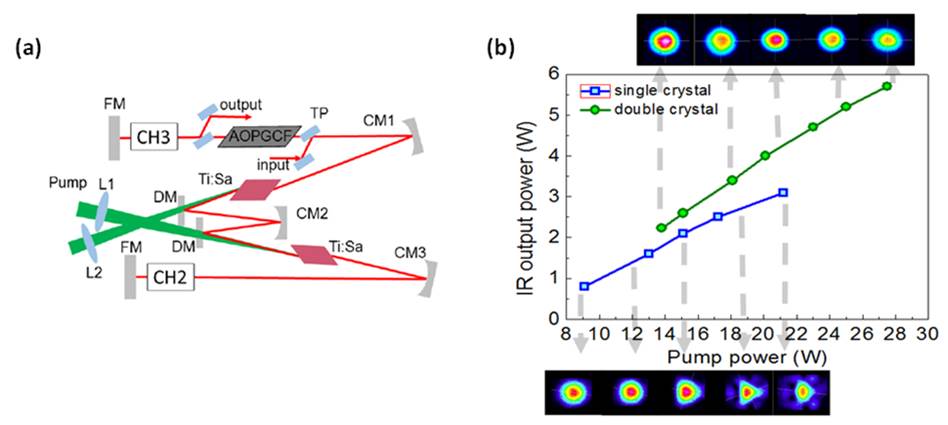
Fig. 1 a) Schematic diagram of two-crystal Ti:Sa amplification. CM: focusing mirrors, L: lenses, DM: dichroic mirror (transparent in the green, reflective in the infrared), TP: fine reflection polarizers, FM: flat cavity mirrors.
b) : Output power versus pump power for a single-crystal (blue curve) and double-crystal (green curve) amplifying cavity. Lower and upper figures: evolution of extracted beam shape as a function of output power: bottom: single-crystal cavity, top: double-crystal cavity.
The excellent stability of the dual-crystal cavity, superior to that of the single-crystal cavity, also enables optimum operation of the acousto-optic dispersive programmable intra-cavity filter (AOPGCF “Mazzler”), whose function is to limit spectral narrowing by reducing the gain at the center of the profile
Depending on the 2 modes, BB or TNB, the pulses from this amplifier can then be amplified by additional stages. On ATTOLab’s new FAB 1/10 laser server, pulses from the first cavity are split into two channels, one remaining at a 10 kHz repetition rate and the other reduced to 1 kHz. Multi-pass amplifiers are then used to achieve powers of 16 W (at 1 or 10 kHz), for a pulse duration of the order of 17.5 fs (Fig. 2b).
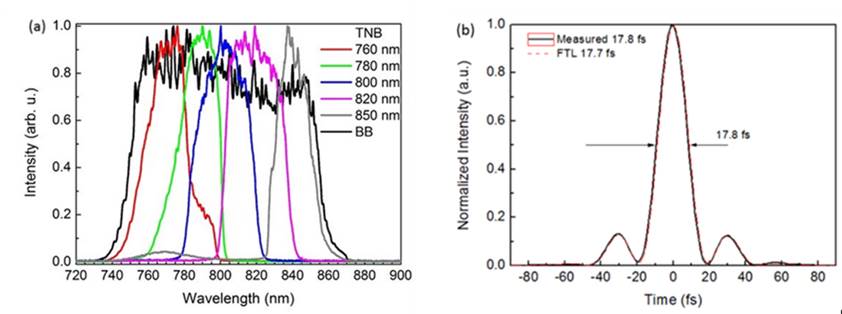
Fig. 2 a) Pulse intensity and spectral width in BB (broad band) mode, and laser pulse intensity in TNB (“Tunable Narrow Band”) mode. b) Right: measured time profile for one pulse
These developments incorporate the constraint of controlling laser pulses on an even shorter time scale, down to the attosecond level (1 as=10-18 s). The temporal width is then only a few optical cycles, and the position of the oscillating field in the pulse’s temporal envelope [8,9], also known as the “carrier-envelope phase”, must be stabilized to ensure that all amplified pulses are identical and reproducible. To this end, a diagnostic system developed in the Impulse laboratory enables rapid measurement of this phase and its adjustment via an analog feedback loop. Residual envelope-carrier phase fluctuations of 250 mrad (100 as) to 350 mrad (150 as) respectively are achieved on each 10 and 1 kHz channel.
The FAB 1-10 laser will thus provide the scientists collaborating on the Equipex Attolab project with all the performance and versatility they need to conduct new experiments on the sub-femtosecond time scale, with world-class laser pulse intensity, duration and stability.
References
D. Strickland & G. Mourou Opt. Com. 56, 219 (1985)
D. F. Hotz. Appl. Opt, 4 527 (1965).
C. A Carter and J. M Harris. Appl. Opt. 23,476 (1984).
A. Golinelli, X. Chen, E. Gontier, B. Bussiere, O. Tcherbakoff, M. Natile, P. D’Oliveira, P.-M. Paul, and J.-F. Hergott. Opt. Lett. 42 2326 (2017).
T. Oksenhendler, D. Kaplan, P.Tournois, G. M. Greetham, and F. Estable. Springer Series in Optical Sciences, 132, 421 (2007).
[6 ] “Ultrabroadband TW-class Ti:sapphire laser system with adjustable central wavelength, bandwidth and multi-color operation.”
A. Trisorio, P. M. Paul, F. Ple, C. Ruchert, C. Vicario, and C. P. Hauri. Opt. Exp. 19 (2011) 20128.
[7 ] “Ti:Sa CEP-Stabilized Laser System Allowing Wavelength Tunability or 1kHz, sub-18fs, TW-class Level Amplification”
A. Golinelli, X. Chen, E. Gontier, B. Bussière, P.-M. Paul, O. Tcherbakoff, P. d’Oliveira, and J.-F. Hergott Laser Congress 2018 (ASSL) OSA Technical Digest (Optical Society of America, 2018), paper AW1A.3
CEP-stabilized, sub-18 fs, 10 kHz and TW-Class 1 kHz dual output Ti: Sa laser with wavelength tunability option
A. Golinelli, X. Chen, E. Gontier, B. Bussière, P.-M. Paul, O. Tcherbakoff, P. d’Oliveira, and J.-F. Hergott
Accepted for publication in Opt. Exp. (2019).
[8 ] “Complete analog control of the carrier-envelope-phase of a high-power laser amplifier.”
C. Feng, J.-F. Hergott, P.-M. Paul, X. Chen, O. Tcherbakoff, M. Comte, O. Gobert, M. Reduzzi, F. Calegari, C. Manzoni, M. Nisoli, and G. Sansone..,
Opt. Exp. 21 25248 (2013).
[9] “Development of an original 10 kHz Ti:Sa regenerative cavity allowing 17 fs CEP stable 1 kHz TW-class amplification or wavelength tunability.”
Thesis A. Golinelli, January 21, 2019 (Manuscript confidential for 5 years).
CEA contact: Jean-François Hergott (LYDIL/SLIC)
Collaboration :
- Groupe Laser Amplitude Technologies – Lisses operations, 2 – 4 rue du Bois Chaland CE 2926, 91029 Evry, France
- CEA-Amplitude Technologies “Impulse” joint laboratory.