The MEC laboratory is a fundamental research lab where two closely related topics are investigated, on the one hand the interaction of matter with a strong laser field, i.e., at high power density, on the other hand the matter at very high energy density, i.e., hot dense plasmas. The MEC lab is composed of 3 interacting research groups, namely Attophysics, Physics at High Intensity (PHI), and High Energy Density Matter (HEDM). The MEC lab comprises 19 permanent scientists and about 15 post-doctorate, PhD and graduate students.
In a strong laser field, corresponding to laser intensity in the range 1014-1019 W/cm2, electrons in atoms, molecules or solids can very efficiently exchange energy with the field or the laser-produced plasma. This efficient field-electron coupling leads to a variety of processes which are investigated in the Attophysics and Physics at High Intensity groups. They mainly develop their experimental programs on the laser facilities of the Saclay Laser-matter Interaction Centre ( SLIC ), partner of the Laserlab-Europe consortium. Facilities include LUCA, PLFA and UHI100 state-of-the-art laser systems.
In the Attophysics group, we study the very rich dynamics of electrons initially bound in atoms and molecules, under strong laser field irradiation (intensity 1014-1015 W/cm2). This dynamics takes place at the ultra-short time scale of one or a few optical cycles, e.g., 2.7 femtosecond at 800 nm wavelength. It governs the multiple ionization and fragmentation of the system, e.g., molecules, the diffraction of electrons, or the spectacular emission of ultra-short light pulses – of typically 100 attoseconds duration – in the extreme-UV range (l = 1 – 100 nm). Such pulses can be used to probe the fastest processes in matter. Moreover, the inelastic scattering of electrons accelerated in a laser field constitutes a very powerful probe of the system itself and of its ultra-fast dynamics, e.g., molecular fragmentation. The probe can combine extreme spatial resolution at the angstrom scale and temporal resolution at the attosecond scale, opening the perspective of following chemical reactions in real time. The Attophysics group develops as well applications of the ultra-short light pulses in the extreme-UV to dynamical studies.
In the Physics at High Intensity group, we study the so-called “ultra- relativistic regime”. To study these new frontiers, occurring upon reflection on overdense plasmas, it is essential to use extremely high temporal-contrast lasers. This opens the way to detailed studies of relativistic plasma optics in well-controlled conditions.
The first topic exploits the extreme nonlinearities induced by the ultra-strong laser fields upon reflection on a plasma, a medium capable of withstanding arbitrarily high laser intensities, in order to efficiently generate trains of energetic attosecond pulses in the XUV domain and isolated attosecond pulses in the UV range.
The second topic is based on ions-laser-acceleration processes. We focus on the fine understanding of various parameters influence such as gradient scale length, laser intensity and polarization and their impact on the ionic acceleration. Special efforts are paid to bring the ion energy and charge toward their maximum limits. This supports a large panel of applications of societal relevance, from the production and diagnosis of dense plasmas to the proton therapy.
The last topic is devoted to the electron acceleration using under-critical (gaseous) targets. Extremely promising laser acceleration of electrons has now been demonstrated up to energy in the GeV range. This concept should decisively contribute to, for example, radiolysis studies at ultra-short time scale, or reducing the dimensions of accelerator-based light sources to the lab scale.
The High Energy Density Matter group studies the atomic physics in hot dense plasmas, i.e., their electronic and radiative properties such as emissivity, opacity, or equation of state. Matter at high energy density appears naturally in most of the astrophysical objects – stars, supernovae -, as well as in laser-produced hot dense plasmas relevant for Inertial Confinement Fusion (ICF). The theory is developed in close connection to experiments at large scale laser facilities, e.g., the LIL (Ligne d’Intégration Laser) and LMJ (Laser MégaJoule), and Z-pinch sources (Sandia).
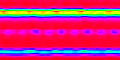